CD90 positive cells exhibit aggressive radioresistance in esophageal squamous cell carcinoma
Introduction
Esophageal cancer is one of the most common malignancies and ranks as the sixth leading cause of cancer-related deaths worldwide, and the major histologic subtype of this disease is esophageal squamous cell carcinoma (ESCC) (1). Normally, patients are asymptomatic during early stages of the disease and are diagnosed at advanced stages with poor prognoses in many cases. The five-year survival rate is only 20% to 30% after curative surgery, but perioperative mortality and postoperative complications occur (2,3). Radiotherapy is a common treatment of patients with locally advanced esophageal cancer (4). To date, intensity-modulated radiotherapy and stereotactic radiotherapy have made significant progress (5). While diagnosis of the disease and radiotherapy technology have improved (6) and the differential effects of radiation dose on tumor and normal tissue have been explored (7), the outcomes remain devastating due to radiation resistance and a limited understanding of its molecular mechanisms (8,9).
Previous studies found cancer was composed of different types of cells, one of which is called cancer stem cells (CSCs). These cells are generally thought to originate from normal stem cells. Therefore, they have similar biological characteristics, such as differentiation, self-renewal capacity, and potential mechanisms for drug resistance, metastasis and recurrence (10).
Recent studies have revealed that CSCs exist not only in acute myeloid leukemia (11) but also in various solid tumors, liver cancer, glioma, etc., further supporting the view that CSCs may represent a valuable potential therapeutic target (12,13).
CD90 is a surface glycoprotein with the molecular weight 25 to 28 kD, and it is expressed on the cytoplasmic membrane of different cell types (14). It is reported that CD90 positive cells from hepatocellular carcinoma cell lines not only display tumorigenic capacity but also exhibit other features of CSCs such as extensive proliferation, differentiation, invasion and metastasis (15). Recent studies also found that CD90 positive cells show some stem cell-like characteristics in ESCCs (16); however, little is known about the underlying molecular mechanisms of how CD90 positive cells promote tumor progression. In this experiment, we explore the differences in the cell biology of radiation sensitivity of CD90 positive cells compared to control cells and attempt to reveal the internal cause of this transformation. Exploration of this novel tumorigenic and metastatic CD90 positive subpopulation in ESCCs may provide an appropriate target for development of effective therapeutic strategies.
Methods
Cell lines and cell culture
The human ESCC cell lines ECA109 and TE13 were obtained from Shanghai Cell Bank (Chinese Academy of Sciences, Shanghai, China) and were cultured in Dulbecco’s modified eagle medium (DMEM) (Gibco, Life Technologies, Carlsbad, CA, USA) supplemented with 10% fetal bovine serum (FBS) (HyClone, GE Healthcare, Little Chalfont, UK) at 37 °C under a humidified atmosphere of 5% CO2.
Construction of CD90 overexpression cells line
Lentiviral particles (Genechem, Shanghai, China) were used to improve the expression level of CD90 in ESCC cell lines. For optimization of transfection conditions with lentiviral vectors, ESCC cells were infected with different of multiplicity of infection (MOI) ratios for 8–10 h in the presence of 5 µg/mL of polybrene. Two days after infection, expression of green florescence protein was measured by fluorescence activated cell sorter (FACSVerse/Calibur/AriaII-SORP, USA) analysis. Our data showed that the infection of sorted cells at an MOI of 100 resulted in an over 80% infection rate without damage. Furthermore, puromycin was used to co-culture at 1 µg/mL for 7–10 days to remove the tumor cells with no transfection according to the manufacturer’s instructions (Figure 1A,B).
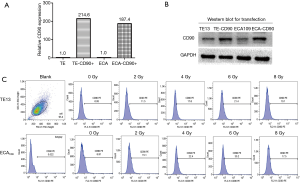
Quantitative real-time RT-PCR
Total cellular RNA was extracted using an RNA extraction kit (TaKaRa, Tokyo, Japan) according to the manufacturer's instructions. Complementary DNA (cDNA) was synthesized with Prime Script TM RT Master Mix (TaKaRa). The qRT-PCR reaction system was prepared following the manufacturer’s instructions and then SYBR®Premix and TaqII (Tli RNaseH Plus) were added to 100 ng cDNA in each test sample, with GAPDH acting as an endogenous control. Gene copy number data analysis was performed according to the 2−ΔΔCt method using GAPDH expression as the control. Each sample was analyzed in triplicate using the StepOne™ Real-Time PCR system (Thermo Fisher Scientific).
Cell viability assay
The cells were seeded in 96-well plates with 5×103 cells/well and then incubated for 24 h of 48 h. Then, 10 µL of Cell Counting Kit-8 solution (CCK-8 Obio Technology, Shanghai, China) was added to each well. The absorbance was measured at a wavelength of 490 nm. The viability of cells was calculated as follows: Viability = (ODtest − ODblank)/(ODcontrol − ODblank) × 100%. All experiments were repeated in triplicate.
Clonogenic survival assay
The exponentially growing cells were trypsinized into single cells and diluted to seed at a specific number of cells (400, 800, 2,000, 4,000, and 6,000) in triplicate in 6-well plates after adhered cells were subjected to 0, 2, 4, 6 and 8 Gy X-rays irradiation at 4.5 Gy/min. After washing the dishes with PBS for 4 h, cells were cultured in complete medium in a 5% CO2 incubator at 37 °C for 14 days, fixed with methanol, and stained with Giemsa. Number of colonies (>50 cells/colony) were counted under the microscope. The experiment was repeated three times.
Flow cytometric analysis
Cells were seeded in 6-well plates at a density of 1×104/L overnight and then exposed to X-rays (6 Gy) while being cultured for 24 h, dissociating samples into single cells which were stained with an Annexin V-APC/7-AAD Apoptosis Kit (Keygene Biotechnology, Nanjing, China) for 15 minutes. Concentrations of antibodies were used according to the manufacturer’s recommendations. Apoptotic cells were detected by flow cytometry with Cell-Quest software (BD Biosciences, San Jose, CA, USA).
Wound-healing migration assay
Different treated cells were seeded in 6-well plates and cultured until reaching 60% confluence, and then the confluent cell monolayer was scratched with a pipette tip to generate a vertical line across the middle of the wells. The degree of wound closure was observed after 0 and 24 h intervals and was photographed by a light microscope.
Transwell invasion assay
Transwell (Corning, MA, USA) plates were used in the invasion assays. Chamber had 8 µm pore size polycarbonate filters and were coated with 2 mg/mL Matrigel basement membrane. A total of 500 µL of medium containing 20% FBS was added to the bottom chamber as chemotactic factor. Then, 1×105 cells of different kinds of treated cells were inoculated into the top chamber with 500 µL DMEM without FBS and cultured for 24 h. The penetrated cells were fixed in basilar membrane with 4% cold paraformaldehyde, stained with 0.1% crystal violet for 15 minutes and washed three times with PBS. The fixed cells were photographed in three random fields with an optical microscope.
Western blot analysis
Total protein from cells was extracted with SDS Lysis Buffer (Keygen, Nanjing, China), and the protein concentration were measured by the BCA kit (Keygen, Nanjing, China). Equivalent amounts of protein from each lysate were separated by SDS-PAGE (10% acrylamide) and then blotted to the PVDF Western blotting membranes (Millipore). Blocked membranes were incubated overnight with primary antibodies. To visualize the immunoblotted proteins, ECL reagents and the Chemidoc XRS imaging system (Quantity One Quantitation software; BioRad Laboratories, Hercules, CA, USA) were used.
Transplantation assay in NOD/SCID mice
For CD90 transplantation studies, CD90 overexpression ESCC cells were injected subcutaneously into the flank of 4- to 5-week-old nonobese diabetic combined immunodeficient (NOD/SCID) mice with 100 µL of a mixture of containing complete medium at a concentration of 105 cells/mL. When the tumor volume exceed 1.0 cm3, a dose of 6 Gy radiation was given one time to the mice, and the animals were observed and recorded for their entire growth cycle for statistical analyses. Each group contained more than 5 animals.
Animal ethical description
The study protocol was conducted and approved by the Animal Ethical and Welfare Committee of Nanjing Medical University of China (Approval No. IACUC-14030109). All participants gave informed consent of the ethical content before doing the animals experiments.
Data analysis
All data are expressed as the mean ± standard deviation (SD) from triplicate assays. Statistical differences between groups were determined using unpaired Student’s t test or ANOVA. All statistical analyses was performed by STATA 11.0 software (StataCorp, College Station, TX, USA) and Prism 5.0 software (GraphPad, La Jolla, CA, USA). P<0.05 was considered to be statistically significant.
Results
Radiation increased CD90 expression in ESCC cells
To study the interaction between radiation and the expression level of CD90 in ESCC cells, two type of ESCC cells (TE13 and ECA109 were treated with a dose of radiation ranged from 0–8 Gy and the expression of CD90 was analyzed in survival cells by flow cytometry. We found that the expression of CD90 kept rising (Figure 1C) and showed statistically significant differences as the radiation dose increased (P<0.05).
CD90 overexpression improved viability after radiation in ESCC cells
To explore if the expression of CD90 affected the survivability of ESCC cells indirectly, a CCK8 assay was used to compare the viability of ESCC cells to normal control and to cells overexpressing CD90 following a dose of 6 Gy radiation for either 24 or 48 h. The results showed that the CD90 overexpressing cells exhibited a significant anti-toxic effect (P<0.05) (Figure 2A,B) after being treated with radiation: the residual CD90 overexpressing cells demonstrated a stronger ability to survive in the toxic effects of radiation compared to the normal control (P<0.001) (Figure 2C,D). These final findings suggested that the overexpression of CD90 further increases the survivability of ESCC cells following radiation.
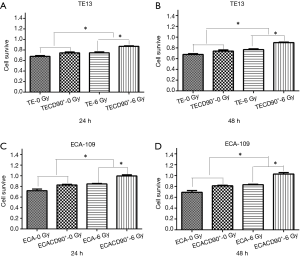
CD90 overexpression reversed the inhibition of radiation on colony forming efficiency
We confirmed CD90 overexpressing tumor cells have better self-renewal capacity (Figure 3A,B). To investigate the effect of CD90 overexpression on radiosensitivity of ESCC cells, normal cells as well as CD90 overexpressing cells were seeded in 6-well plates and then exposed to radiation ranging from 0–8 Gy. The results showed a significant improvement in the colony forming ability of the CD90 overexpressing cells compared to the control group after irradiation, indicating that radiation inhibits the growth of tumor cells, but the gene of CD90 can partly reverse the effects. Moreover, the SF data were fitted into the single-hit multi-target model formula: SF =1−(1−e−D/D0)n (Figure 3C,D). The results showed that SF2 was 0.61 and 0.73 for TE13 cells and 0.66 and 0.79 for ECA109 cells.
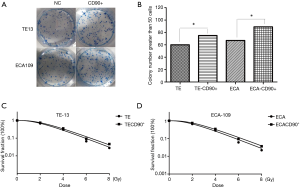
CD90 overexpression inhibited radiation–induced apoptosis in ESCC cells
It is reported that radiation play an independent role in the cell apoptosis process of a tumor (17). To explore the different apoptosis rates of the CD90 overexpressing and normal control groups, all cells were exposed to radiation of 0 and 6 Gy. We then detected the early apoptosis proportion by flow cytometric analysis. This trial showed that radiation promoted the apoptosis of ESCC cells (P<0.05), which is similar to previously reported results(18); however, cells with high expression of CD90, a candidate marker of CSCs, exhibited a significant inhibition of radiation-induced apoptosis (P<0.001) (Figure 4).
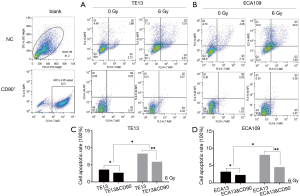
CD90 overexpression enhanced radiation–induced migration and invasion in ESCC cells
Previous studies reported that the ability of migration and invasion increased with irradiation in esophageal cancer cells (19). We evaluated the migration distance of ESCC cells using a wound-healing assay in vitro. The results showed that the CD90 overexpressing cells closed the wound area significantly quicker than the control group (P<0.05) (Figure 5); when treated with a radiation dose of 6 Gy, the migration ability was further strengthened (P<0.05). Similarly, the invasion ability also greatly increased in irradiated CD90 overexpressing cells (Figure 4). All of this data suggested that the overexpression of CD90 further improves the ability of migration and invasion of ESCC cells.
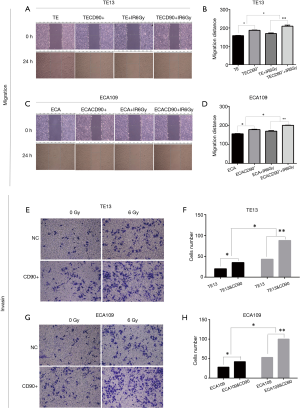
CD90 overexpression prompted radiation-induced EMT in ESCC cells
Previous studies in vitro found that radiation-induced EMT changes have been discovered in colon cancer (20), breast cancer (21), lung cancer, etc. To further study the radiation-induced EMT-associated changes in ESCC cells, we compared the differences in varying protein levels of the CD90 overexpressing and control cells after irradiation. We found the classical epithelial marker E-cadherin was significantly downregulated, while the mesenchymal marker vimentin was upregulated after irradiation (22). This included the expression of EST-1 and downstream signaling pathways involving MMPs, such as MMP2 and MMP10 (16). It has been suggested that EMT pathways are activated more significantly in the CD90 overexpression group, which may help explain the enhanced migration, invasion, and anti-apoptotic capabilities of ESCC cells (Figure 6A,B).
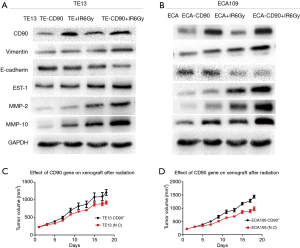
CD90 overexpression improved the radiation resistance of ESCC cells in vivo
To investigate the radiosensitivity between the CD90 overexpressing and control cells in vivo, these cells were subcutaneously implanted in severe combined immunodeficiency (SCID) mice. When the tumor volume exceeded 1.0 cm3, a single dose of 6 Gy radiation was administered to the mice, and the animals were observed and recorded for their entire growth cycle for statistical analysis. Our data revealed that the growth efficiencies of the tumors in mice injected with CD90 overexpressing cells were higher than those of mice injected with control cells and that the CD90 overexpression cells showed significantly higher irradiation resistance (Figure 6C,D).
Discussion
ESCC is a malignancy with a high cancer-related mortality rate; the outcome of traditional treatment is unsatisfactory, suggesting a need for innovation (23). To date, the hypothesis of CSCs in solid tumors has been widely accepted, including colorectal, breast, and head and neck cancers, and CSCs have been shown to play a major role in recurrence and metastasis of cancer. Targeting CSCs will be a potential promising therapeutic strategy for selective neoplasms; however, research of CSCs in ESCC is relatively rare.
CD90 has been identified as a marker for CSC in many tumors, including liver cancer (15) and glioma (24), but its potential role in ESCC has not been completely characterized. Previous studies have shown that the expression of CD90 is normal in premalignant dysplastic tissues (i.e., approximately 0 to 1.4%), while its expression ranges from 2.4% to 10% in ESCCs (16). This suggested that CD90 expression may be associated with tumor heterogeneity and malignancy.
In this study, we found that CD90 expression levels in ESCC cells increased as radiation dose increased. This may be caused by irradiation removing a large number of tumor cells with the remaining cells exhibiting a higher proportion of radiation-resistant CSCs, further supporting a relationship between CD90 and CSCs in ESCC cells.
In addition, the results showed that the expression of CD90 in residual ESCC cells gradually increased with increases in the radiation dose; however, it suddenly declined when the radiation dose was increased to 8 Gy. Thus, we speculate that this was the limit for a single radiation treatment, so we used a dose of 6 Gy for this study. We compared the biological changes of CD90 overexpressing and control cells before and after irradiation. The data revealed that cells overexpressing CD90 exhibited increased cell survival, proliferation, invasion, and migration, and these cells showed significant resistance to radiation.
To explore the real mechanism of how CD90 plays a role in ESCCs after irradiation, we studied the EMT pathway, which not only plays an important role in tumorigenicity and chemotherapy resistance but also increases some properties of CSCs when activated by radiation (25,26). Our data showed that ESCC cells with overexpression of CD90 had increased the colony forming rate, migration and invasion, and inhibition of apoptosis after irradiation, partly due to the activation of the EST-1 gene and its downstream MMPs, which are involved in the classic EMT pathway (27,28). In addition, the changes in gene expression of vimentin and E-cadherin suggested that the overexpression of CD90 in ESCC cells may promote malignant subtypes and the associated poor prognosis and increased resistance after irradiation (29).
We believe that this is the first study to confirm the relationship between the CD90 gene and radiosensitivity in ESCC cells. Furthermore, the findings revealed in this trial may be partly explained by the observation that ESCCs tend to more readily exhibit recurrence and metastasis after radiotherapy in patients with high expression of CD90. However, our research is limited to cell lines TE13 and ECA109; another deficiency is that this trial was not related to clinical specimens (30-32).
Conclusions
Our findings revealed that the effect of CD90 overexpression in ESCC cells was complicated and at least partially mediated by an alteration of Ets-1 transcription and its downstream matrix MMP targets to activate the EMT pathway, which exhibit the capability for greater tumorigenicity, invasion, metastasis and radiotherapy resistance. Therefore, a high expression of CD90 may enhance the ability to initiate and maintain tumor growth, further promoting tumor invasion and distant metastasis in patients with ESCC.
Therefore, the CD90 gene will likely be a new therapeutic target for such special populations with radioresistance. Research on the mechanism of CD90 may provide a new perspective to therapeutic strategies for patients with ESCC.
Acknowledgements
Funding: This work was supported by the Natural Science Foundation of China (No. 81472809, No. 81672983), Innovation Team [No. LJ201123 (EH11)], A Project Funded by the Priority Academic Program Development of Jiangsu Higher Education Institutions (PAPD) (JX10231801), and grants from Key Academic Discipline of Jiangsu Province “Medical Aspects of Specific Environments”.
Footnote
Conflicts of Interest: The authors have no conflicts of interest to declare.
Ethical Statement: The study protocol was conducted and approved by the Animal Ethical and Welfare Committee of Nanjing Medical University of China (Approval No. IACUC-14030109). All participants gave informed consent of the ethical content before doing the animals experiments.
References
- Yu S, Yang CS, Li J, et al. Cancer Prevention Research in China. Cancer Prev Res (Phila) 2015;8:662-74. [Crossref] [PubMed]
- Jemal A, Bray F, Center MM, et al. Global cancer statistics. CA Cancer J Clin 2011;61:69-90. [Crossref] [PubMed]
- Parkin DM, Bray FI, Devesa SS. Cancer burden in the year 2000. The global picture. Eur J Cancer 2001;37 Suppl 8:S4-66. [Crossref] [PubMed]
- Fokas E, Rodel C. Definitive, Preoperative, and Palliative Radiation Therapy of Esophageal Cancer. Viszeralmedizin 2015;31:347-53. [Crossref] [PubMed]
- Lau SK, Zhao X, Carmona R, et al. Frameless single-isocenter intensity modulated stereotactic radiosurgery for simultaneous treatment of multiple intracranial metastases. Transl Cancer Res 2014;3:383-90. [PubMed]
- Kumar R, Korideck H, Ngwa W, et al. Third generation gold nanoplatform optimized for radiation therapy. Transl Cancer Res 2013.2. [PubMed]
- Prasanna PG, Stone HB, Wong RS, et al. Normal tissue protection for improving radiotherapy: Where are the Gaps? Transl Cancer Res 2012;1:35-48. [PubMed]
- D'journo XB, Thomas PA. Current management of esophageal cancer. J Thorac Dis 2014;6 Suppl 2:S253-64. [PubMed]
- Best LM, Mughal M, Gurusamy KS. Non-surgical versus surgical treatment for oesophageal cancer. Cochrane Database Syst Rev 2016;3:D11498. [PubMed]
- Visvader JE, Lindeman GJ. Cancer stem cells in solid tumours: accumulating evidence and unresolved questions. Nat Rev Cancer 2008;8:755-68. [Crossref] [PubMed]
- Nishida H, Yamazaki H, Yamada T, et al. CD9 correlates with cancer stem cell potentials in human B-acute lymphoblastic leukemia cells. Biochem Biophys Res Commun 2009;382:57-62. [Crossref] [PubMed]
- Ranji P, Salmani KT, Saeedikhoo S, et al. Targeting cancer stem cell-specific markers and/or associated signaling pathways for overcoming cancer drug resistance. Tumour Biol 2016;37:13059-75. [Crossref] [PubMed]
- Ishiwata T. Cancer stem cells and epithelial-mesenchymal transition: Novel therapeutic targets for cancer. Pathol Int 2016;66:601-8. [Crossref] [PubMed]
- Barker TH, Hagood JS. Getting a grip on Thy-1 signaling. Biochim Biophys Acta 2009;1793:921-23.
- Yang ZF, Ho DW, Ng MN, et al. Significance of CD90+ cancer stem cells in human liver cancer. Cancer Cell 2008;13:153-66. [Crossref] [PubMed]
- Tang KH, Dai YD, Tong M, et al. A CD90(+) tumor-initiating cell population with an aggressive signature and metastatic capacity in esophageal cancer. Cancer Res 2013;73:2322-32. [Crossref] [PubMed]
- Lee CL, Blum JM, Kirsch DG. Role of p53 in regulating tissue response to radiation by mechanisms independent of apoptosis. Transl Cancer Res 2013;2:412-21. [PubMed]
- Ge Y, Gu X, Wang B. Analysis of esophageal cancer cell lines exposed to X-ray based on radiosensitivity influence by tumor necrosis factor-alpha. J Xray Sci Technol 2016;24:761-9. [Crossref] [PubMed]
- He E, Pan F, Li G, Fractionated Ionizing Radiation Promotes Epithelial-Mesenchymal Transition LI J. in Human Esophageal Cancer Cells through PTEN Deficiency-Mediated Akt Activation. PLoS One 2015;10:e126149.
- Diao WL, Luo L, Luo Q. Effect of X-ray irradiation on epithelial-mesenchymal transition of colorectal cancer SW480 cells. J Biol Regul Homeost Agents 2016;30:553-58. [PubMed]
- Yuan W, Yuan Y, Zhang T, et al. Role of Bmi-1 in regulation of ionizing irradiation-induced epithelial-mesenchymal transition and migration of breast cancer cells. PLoS One 2015;10:e118799. [PubMed]
- Zhang H, Luo H, Jiang Z, et al. Fractionated irradiation-induced EMT-like phenotype conferred radioresistance in esophageal squamous cell carcinoma. J Radiat Res 2016;57:370-80. [Crossref] [PubMed]
- Ke L. Mortality and incidence trends from esophagus cancer in selected geographic areas of China circa 1970-90. Int J Cancer 2002;102:271-74. [Crossref] [PubMed]
- He J, Liu Y, Zhu T, et al. CD90 is identified as a candidate marker for cancer stem cells in primary high-grade gliomas using tissue microarrays. Mol Cell Proteomics 2012;11:M111.010744.
- Kalluri R, Weinberg RA. The basics of epithelial-mesenchymal transition. J Clin Invest 2009;119:1420-28. [Crossref] [PubMed]
- Polyak K, Weinberg RA. Transitions between epithelial and mesenchymal states: acquisition of malignant and stem cell traits. Nat Rev Cancer 2009;9:265-73. [Crossref] [PubMed]
- Mukherjee T, Kumar A, Mathur M, et al. Ets-1 and VEGF expression correlates with tumor angiogenesis, lymph node metastasis, and patient survival in esophageal squamous cell carcinoma. J Cancer Res Clin Oncol 2003;129:430-36. [Crossref] [PubMed]
- Mukherjee S, Roth MJ, Dawsey SM, et al. Increased matrix metalloproteinase activation in esophageal squamous cell carcinoma. J Transl Med 2010;8:91. [Crossref] [PubMed]
- Hassan H, Greve B, Pavao MS, et al. Syndecan-1 modulates beta-integrin-dependent and interleukin-6-dependent functions in breast cancer cell adhesion, migration, and resistance to irradiation. FEBS J 2013;280:2216-27. [Crossref] [PubMed]
- Goossens N, Nakagawa S, Sun X, et al. Cancer biomarker discovery and validation. Transl Cancer Res 2015;4:256-69. [PubMed]
- Berger NA. Actionable Intelligence Provided by Pancreatic Cancer Genomic Landscape: Are Targets for Curative Therapy On The Map? Transl Cancer Res 2016;5:S243-7. [Crossref] [PubMed]
- van Rossum PS, Xu C, Fried DV, et al. The emerging field of radiomics in esophageal cancer: current evidence and future potential. Transl Cancer Res 2016;5:410-23. [Crossref]