Clinical application of a surgical navigation system based on virtual thoracoscopy for lung cancer patients: real time visualization of area of lung cancer before induction therapy and optimal resection line for obtaining a safe surgical margin during surgery
Introduction
Induction therapy followed by surgical treatment is sometimes performed and considered as a valid treatment option for lung cancer patients complicated with chest wall invasion (1,2). Induction therapy has been reported to be useful for eradicating micro-metastasis, sparing vital structures, obtaining a safe and secure surgical margin, and extracting occult metastasis by prolonging surgical treatment, and is associated with improved compliance in comparison to adjuvant therapy. However, surgeons have not been able to grasp ‘the area of the tumor before induction therapy’ or ‘the distance between the resection line and the edge of the original tumor’ intraoperatively because the effectiveness of induction therapy makes the original area of the tumor ‘invisible’.
We have developed a surgical navigation system that presents virtual thoracoscopic images [using high resolution computed tomography (HRCT) image data], as if you are observing the intra-thoracic cavity in synchronization with the real thoracoscopic view. This system also enables the visualization of the tips of the forceps on the virtual image. This system enabled the ‘area of lung cancer before induction therapy’ and the ‘optimal resection line for obtaining a safe surgical margin’ to be visualized as a virtual thoracoscopic view in synchronization with the real thoracoscopic view. We applied our navigation system in the clinical setting, in the treatment of two lung cancer patients with chest wall invasion after induction therapy.
Methods
Patients
In 2017, 10 lung cancer patients with chest wall invasion received surgical treatment at Nagoya University Hospital. Our surgical navigation system was applied in the treatment of 2 of the 10 patients; these patients were enrolled in the study (Table 1). The patients also received induction chemoradiotherapy prior to surgical treatment. All information on radiological and pathological variables was collected from the patients’ medical records. This study protocol was approved by the institutional review board of the university hospital (UMIN000020820).
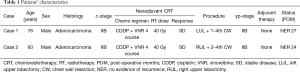
Full table
Concept of our surgical navigation system
Our surgical navigation system was produced with the aim of visualizing the ‘area of lung cancer before induction therapy’ and the ‘optimal resection line for obtaining a safe surgical margin’ as a virtual thoracoscopic view in synchronization with the real thoracoscopic view. This navigation system consisted of three-dimensional (3D) positional tracker and a virtual thoracoscopy system. The 3D positional tracker was used to recognize the positional information of the thoracoscope. The virtual thoracoscopy system generated the virtual thoracoscopic views based on HRCT image data. Combined with these two technologies, patient-to-image registration was performed; the results showed that virtual thoracoscopic views were generated in synchronization with the real thoracoscopic view. The system configuration is shown in Figure 1A.
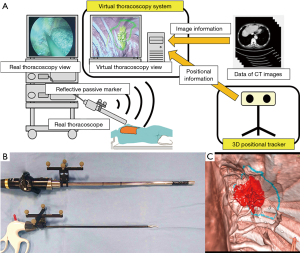
Based on previous reports that the optimal surgical margin was over 2 cm for reducing the risk of local recurrence (3,4), we considered that a safe surgical margin should be a distance of >2 cm from the tumor. Thus, optimal resection line was indicated on this virtual thoracoscopic view at a distance of 2 cm from the area of the original tumor. The primary end-point of this study was the achievement of a pathologically negative surgical margin.
Treatment strategy using our surgical navigation system
Treatment was performed in the following steps:
- STEP 1: HRCT scans of the enrolled patients were obtained prior to any treatment.
- STEP 2: To emphasize the important anatomical structures in the virtual thoracoscopic view, segmentation was performed using the HRCT data. The area of lung cancer before induction therapy and the optimal resection line for obtaining a safe surgical margin were extracted and drawn on the virtual thoracoscopic view.
- STEP 3: The surgical navigation system was started via two-port video-assisted thoracic surgery (VATS), prior to surgical treatment. The patient-to-image registration was performed for the synchronization between real and virtual thoracoscopic view, thereafter we were able to see real and virtual thoracoscopic view in synchronization which also indicated ‘area of lung cancer before induction therapy’ and ‘optimal resection lines for obtaining a safe surgical margin’.
- STEP 4: Landmarks for the optimal resection line on the chest wall, which were determined using a surgical navigation system, were engraved using an electric scalpel attached to a forcep.
- STEP 5: Lobectomy combined with chest wall resection via thoracotomy was performed using the landmarks of the optimal resection line to obtain a sufficient surgical margin.
Positional information
We use the Polaris Spectra optical tracking system (NDI, Warterloo, Ontario, Canada) as a 3D positional tracker. The Polaris system enabled the position of a thoracoscope or forceps to be detected using reflective passive markers that were attached to the respective instruments (Figure 1B). We established the relationship between the attached reflective passive markers and the real thoracoscope or forceps tip in advance of the surgical navigation system using the NDI 6D Architect (NDI, Waterloo, Ontario, Canada) software program. The positional information was continuously sent to the virtual thoracoscopy system during navigation.
Patient-to-image registration
To generate virtual thoracoscopic views synchronized with real thoracoscopic views, the navigation system requires thoracoscope camera position in the preoperative HRCT image coordinate system. The positional information of the thoracoscope and forceps is measured by the 3D positional tracker. Therefore, we transform the positions of the thoracoscope and forceps in the 3D positional tracker coordinate system into their positions in the preoperative HRCT image coordinate system using patient-to-image registration. The patient-to-image registration computes a transformation matrix from the 3D positional tracker coordinate system to the preoperative HRCT image coordinate system. We use the point pairs matching registration method to obtain this transformation matrix.
We selected six or more anatomical landmarks as fiducials for registration. These landmarks were located at some anatomical points of the chest (e.g., on the external body or intra thoracic cavity) and were recognized via the 3D positional tracker. The navigation system performed patient-to-image registration using the positional information of these fiducials.
Virtual thoracoscopic views
The proposed navigation system generated virtual thoracoscopic views using preoperative HRCT images and was based on the virtual endoscopy system introduced by Mori et al. (5). Virtual endoscopy visualizes the human body based on medical images of hollow organs as a simulated endoscopic view. The virtual thoracoscopic views could be visualized in real time using the fast volume rendering method by exchanging data from multiple CT images (6). To emphasize important anatomical structures in the virtual thoracoscopic view (e.g., the original area of the tumor or the optimal surgical resection line), the proposed navigation system allowed the segmented anatomical regions to be highlighted with different colors (Figure 1C). These specific anatomical structures were manually segmented by tracing HRCT images or virtual thoracoscopic views prior to surgery.
The proposed navigation system provides virtual thoracoscopic views synchronized with the real thoracoscopic views after the patient-to-image registration. The navigation system then obtains the positions of the thoracoscope and forceps in the preoperative HRCT image coordinate system by transforming the positions of the thoracoscope and forceps measured in the 3D positional tracker using the transformation matrix calculated in the patient-to-image registration. The virtual endoscopy in the navigation system generates virtual thoracoscopic views that correspond to the real thoracoscope positions using transformed positional information.
The evaluation of registration accuracy
Registration error was defined as a difference between ‘the distance from one point of the optimal resection line to the nearest edge of tumor after induction therapy in the virtual thoracoscopic view’ and ‘the distance from a landmark engraved by an electric scalpel to the nearest edge of the tumor on the resected specimen’.
Results
Our surgical navigation system was applied in the treatment of two patients who had lung cancer complicated by chest wall invasion. They received induction chemotherapy combined with concurrent radiotherapy prior to surgical resection (Figure 2). We planned upper lobectomy combined with chest wall resection. The operations were started with two-port VATS, and the surgical navigation system was activated at the same time (Figures 3,Video 1). After registration between each anatomical landmark and the fiducials of the 3D-CT image data, the virtual thoracoscopic view synchronized with the real thoracoscopic view. The virtual thoracoscopic view also simultaneously indicated the ‘area of lung cancer before induction therapy’ and ‘optimal resection lines for obtaining a safe surgical margin’. Before thoracotomy, we marked the optimal lines using an electric scalpel (Figure 3B). These landmarks allowed us to perform lobectomy and chest wall resection with a sufficient surgical margin. A pathological examination revealed that the surgical margin was negative.
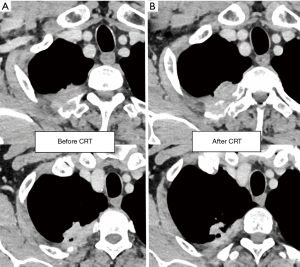
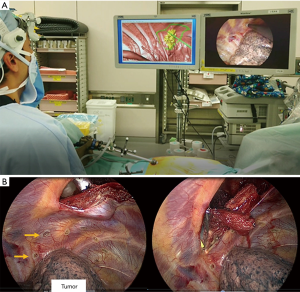
The mean time for using surgical navigation system was 22 min. The registration error in cases 1 and 2 was 0.9 and 0.6 cm, respectively. No complications related to the navigation system were encountered during or after the procedures.
Discussion
Our surgical navigation system was successfully applied in the clinical setting in the treatment of two lung cancer patients with chest wall invasion. This system visualizes the ‘area of lung cancer before induction therapy’ and the ‘optimal resection line for obtaining a safe surgical margin’ in synchronization with a real thoracoscopic view. These provisions improved the surgeon’s confidence by ensuring that a sufficient surgical margin was attained.
Induction therapy followed by surgical treatment is sometimes considered for patients with locally advanced lung cancer and is widely accepted as an effective treatment strategy (1,2). The recent development of new drugs for lung cancer (e.g., immune checkpoint inhibitors and molecular targeted therapy) has markedly improved the prognosis of patients. The number of salvage surgery operations for patients with advanced-stage lung cancer may increase with the combined use of these new agents in induction therapy. There are two important issues that the surgeon must remember when treating patients who have undergone induction therapy. First, the surgeon must consider the tissue vulnerability of the intrathoracic organs and must operate more carefully than usual. Second, is that the original area or position of the tumor may be difficult to grasp visually, because of the effectiveness of induction therapy. The disappearance of the tumor may cause surgeons to hesitate or become anxious when making decisions regarding the surgical resection line. Our surgical navigation system may be a solution to this problem.
The clinical application of intraoperative surgical navigation systems based on CT image data has already been reported, especially in the neurosurgery and gastrointestinal surgery fields (7-10). Hayashi et al. reported the clinical application of a surgical navigation system for gastric surgery (11). Their navigation system could provide a virtual laparoscopic view of the patient anatomy using preoperative HRCT image data; the virtual laparoscopic view was provided in real time beside the real laparoscopic view during surgery. As a result, their navigation system enabled the accurate identification of vascular anatomy, which could be used intraoperatively as a guide for gastrectomy. Their technique was applied in our navigation system for thoracic surgery. This system which used preoperative HRCT image data to create a virtual thoracoscopic view, could be applied in the clinical setting. Furthermore, our navigation system could provide additional information in the virtual thoracoscopic view that was not visible in the real thoracoscopic view.
Surgical navigation systems have not been employed in the thoracic surgery field because positional information can be unstable and may not be detected by the system in the thoracic cavity. The lungs and most neighboring structures are not fixed and are easily displaced during surgery by the beating heart or the collapse of the lung due to differential lung ventilation as a result of the operation. The current surgical navigation system was applied for patients with lung cancer complicated by chest wall invasion, because the tumors were fixed to their chest walls. This system could also be useful for improving a surgeon’s confidence of obtaining a sufficient surgical margin in the treatment of lung cancer patients who have not received induction therapy or for patients with mediastinal tumors. We plan to extend the clinical application of our navigation system to such patients. Furthermore, in patients with other cancer, when tumors are fixed or have invaded their body walls, the current navigation system can be applied to surgery for other organs. After accumulating a number of lung cancer patients, we are planning to apply this system to patients with other cancers.
We recognized the need to make this surgical navigation system more accurate and reliable for thoracic surgeons; thus, the correction of the registration error and positional deviation are important tasks.
In conclusion, this surgical navigation system allowed us to obtain a ‘CT-derived virtual intra-thoracic 3D view of the patient’ that was aligned and synchronized with the real thoracoscopic view. This system, which can identify areas of cancer invasion before induction therapy, might be useful for deciding optimal surgical resection lines.
Acknowledgments
Funding: This work was supported by JSPS KAKENHI Grant Number 15K19933 Grant-in-Aid for Young Scientists (B).
Footnote
Conflicts of Interest: The authors have no conflicts of interest to declare.
Ethical Statement: The authors are accountable for all aspects of the work in ensuring that questions related to the accuracy or integrity of any part of the work are appropriately investigated and resolved. This study protocol was approved by the institutional review board of the university hospital (UMIN000020820) and written informed consent was obtained from all patients.
Open Access Statement: This is an Open Access article distributed in accordance with the Creative Commons Attribution-NonCommercial-NoDerivs 4.0 International License (CC BY-NC-ND 4.0), which permits the non-commercial replication and distribution of the article with the strict proviso that no changes or edits are made and the original work is properly cited (including links to both the formal publication through the relevant DOI and the license). See: https://creativecommons.org/licenses/by-nc-nd/4.0/.
References
- Yokoi K, Taniguchi T, Usami N, et al. Surgical management of locally advanced lung cancer. Gen Thorac Cardiovasc Surg 2014;62:522-30. [Crossref] [PubMed]
- Kawaguchi K, Yokoi K, Niwa H, et al. A prospective, multi-institutional phase II study of induction chemoradiotherapy followed by surgery in patients with non-small cell lung cancer involving the chest wall (CJLSG0801). Lung Cancer 2017;104:79-84. [Crossref] [PubMed]
- Sawabata N, Ohta M, Matsumura A, et al. Optimal distance of malignant negative margin in excision of nonsmall cell lung cancer: a multicenter prospective study. Ann Thorac Surg 2004;77:415-20. [Crossref] [PubMed]
- Wolf AS, Swanson SJ, Yip R, et al. The impact of margins on outcomes after wedge resection for stage I non-small cell lung cancer. Ann Thorac Surg 2017;104:1171-8. [Crossref] [PubMed]
- Mori K, Urano A, Hasegawa J, et al. Visualized endoscope system-an application of virtual reality technology to diagnostic aid. IEICE Trans Inf Syst 1996;E79-D:809-19.
- Mori K, Suenaga Y, Toriwaki J. Fast volume rendering based on software optimization using multimedia instructions on PC platform. CARS 2002. Berlin: Springer, 2002:467-72.
- Gumprecht H, Trost HA, Lumenta CB. Neuroendoscopy combined with frameless neuronavigation. Br J Neurosurg 2000;14:129-31. [Crossref] [PubMed]
- Busse H, Schmitgen A, Trantakis C, et al. Advanced approach for intraoperative MRI guidance and potential benefit for neurosurgical applications. J Magn Reson Imaging 2006;24:140-51. [Crossref] [PubMed]
- Ieiri S, Uemura M, Konishi K, et al. Augmented reality navigation system for laparoscopic splenectomy in children based on preoperative CT image using optical tracking device. Pediatr Surg Int 2012;28:341-6. [Crossref] [PubMed]
- Takiguchi S, Fujiwara Y, Yamasaki M, et al. Laparoscopic intraoperative navigation surgery for gastric cancer using real-time rendered 3D CT images. Surg Today 2014;45:618-24. [Crossref] [PubMed]
- Hayashi Y, Misawa K, Oda M, et al. Clinical application of a surgical navigation system based on virtual laparoscopy in laparoscopic gastrectomy for gastric cancer. Int J Comput Assist Radiol Surg 2016;11:827-36. [Crossref] [PubMed]