Air leak after lung resection: pathophysiology and patients’ implications
Air leaks: where we are
Management of air leaks after thoracic surgery remains a critical aspect in the postoperative course of patients following lung resection. The objective of this paper is to focus on aspects often neglected by surgeons but at the basis of new research line and prospective.
Chest tube duration has been recognized as one of the main causes of prolonged hospital stay, impacting therefore both on hospital finances and patient quality of life (1). What arises most form the analysis made by Varela and colleagues was in fact, the difficulty to conduct cost-effectiveness analyses of surgical strategies to avoid the prolonged air leak.
Furthermore, in a recent paper from Salati, it was evident as the occurrence of a pneumothorax after discharge was one of the most frequent reasons for readmission defined as a re-hospitalization for any cause related to the operation within 30 days after discharge (2). We cannot exclude that pneumothorax were due to a latent recurrent air leak. However, they were not able to find differences in the readmission rate for pneumothorax of the two matched groups after the implementation of a fast track policy. This evidence confirms the general attitude to update the definition of air leak: nowadays the limit of 5 days appears to be more suitable for VATS procedures and fast-track units.
In recent findings from our group of Ancona, 13% of the patients submitted to pulmonary major resections had at least one air leak recurrence occurring between 4 and 11 h after the initial stop of air leaking (3). In this group of 129 patients, a larger proportion of patients with COPD developed recurrent air leak, suggesting a more precautionary approach in deciding to remove the chest tube in the first 24 hours, even in the case of absence or cessation of air leak.
Obviously, intermittent or recurrent air leaks detectable during instantaneous assessment with traditional chest drainage system have contributed also to increase interobserver variability in deciding when to remove the chest tube (4). A high rate of disagreement related to the indication to remove or clamp chest tubes after lung resection has been in the last years overcomes with the use of the electronic chest drainages. However, the underlying mechanism of a prolonged air leak or recurrences and its possible preventions needs to be further investigated from a physiology point of view.
Up to now we have only indirect instrument to deal with air leaks. They are represented by measures to prevent it, measure to predict it like scoring systems, and measure to indirectly treat it, like endobronchial valves. However, only investigating the real pathophysiology underneath an air leak we can develop ad-hoc treatments.
Patient experience of air leak-related complications
If the postoperative complications, such as a prolonged air leak, may have a relevant impact on quality of life following surgery, has been object of some investigations. However, the patient’s perception of a surgical complication remains subjected to many factors not easily detectable and not related to the entity of the objective clinical problem.
What Lackey perfectly emphasizes in his review, has to be considered every time we are dealing with a postoperative complication, which is delaying the discharge of a patients and affecting the quality of care delivered (5). Patients are more concerned with long-term functional status after the operation rather than immediate surgical morbidity. And every time the surgeon have a preoperative consultations with these patients the main questions is always if they would be able to resume an acceptable daily lifestyle.
Experiencing a prolonged air leak for a patients and surgeons is a “long term complication” and patients need to learn dealing with it also at home, modifying completely their quality of life.
However, our recent research on 171 patients submitted to pulmonary resections found that patients with longer hospital stay or experiencing postoperative complications perceived a similar quality of care compared with those with a regular postoperative course. This is a further confirmation that patients reported outcomes are not surrogated of clinical outcomes. However, the study didn’t explore the effect of the air leak or other main complications after the discharge from hospital on their home daily lifestyle (6).
In the last decades thoracic surgeons are trying to improve the quality of life of patients with prolonged air leak, discharging, when safe, people at home with portable chest drainage. A recent English paper demonstrated 772 bed-days saved (GBP 270,000 cost-saving) in 20 patients discharged at home with chest portable drainage for air leak (7). Rieger and colleagues in 2007 already showed that, using a portable device for the outpatient management of select patients with excessive fluid or air leaks, resulted in hospital cost reduction, improving patient satisfaction by allowing earlier discharge and full mobilization (8).
Definition of air leaks and scoring systems
Figures from the last edition of the European Society of Thoracic Surgeons Database Annual report showed that the incidence of an air leak lasting longer than five days is about 8.6% after lobectomy, 6.7% after segmentectomy and 3.5% after wedge resection. The entity of the problem seems to be more accurate with the increasing use of digital drains.
The impact of air leak and the prolonged hospital stay, lead authors to predict the occurrence of this complication in an effort to identify high-risk patients in whom to apply preventative measures.
The most consistently reported risk factors are reduced pulmonary function indicative of a damaged and fragile lung parenchyma, upper lobe resections, low body mass index and presence of pleural adhesions (9). Brunelli and coll (10) have recently developed an aggregate risk score stratifying the risk of PAL and based on four weighted factors: age>65, 1 point; presence of pleural adhesions, 1 point; FEV1 <80%, 1.5 points; body mass index <25.5, 2 points.
According to the score, 4 risk classes were derived with an incremental risk of PAL: the risk of PAL was 0 in class A (no risk factors present, score 0), 6.7% in class B (score 1), 10.9% in class C (score 1.5–3), and 25.7% in class D (>3.5).
Orsini and colleagues (11) have recently validated another score for PAL, the so called index for prolonged air leak (IPAL), previously developed from the French national Thoracic Surgery registry (Epithor) (12), in a population of patients having received VATS anatomical lung resections. The IPAL is a risk model compounded by seven variables associated with air leak longer than 7 days: gender, dyspnoea score, type of lung resection, location of the resection, pleural adhesions and low BMI. In this population the IPAL score showed a satisfactory predictive capability with a ROC curve of 0.72.
Standardization of terminology
With the intent to standardize terminology and nomenclature on the subject of management of pleural space after lung resection, four major European and North American Thoracic Surgery Organizations (ESTS, AATS, STS and GTSC) have published a joint position paper (13).
Authors attempted to clarify the terminology related to the application of suction to the chest drains:
- − “passive drainage”: occurs when the intrapleural pressure rises above the atmospheric pressure;
- − “active drainage”: occurs when a subatmospheric pressure (negative) is applied to the pleural space either by an external pump or by creating a column of liquid within the chest tube that extends below the level of the pleural space (siphoning effect).
Recent findings have shown large variations of values of pleural pressure measured during the last hour before chest tube removal in a series of uncomplicated pulmonary lobectomies, while the chest tube was maintained on water seal without external suction (14). Pressures can vary from positive values to values as negative as minus 40 cmH2O in the same patients and during a relatively short period of time.
To clarify terminology in the context of “active drainage”, the situation where an external suction is applied to the drain has been defined as “external suction applied”. In all other cases the definition “no external suction applied” has been implemented.
Another important definition is the distinction between regulated (variable) suction and unregulated (fixed) suction. Regulated suction is a form of active drainage obtained through the application of an external source of suction capable of modifying its level of negative pressure in response to variations of pressure inside the pleural space with the aim to maintain a pre-set value of pressure. Non-regulated or fixed suction is a form of active drainage provided by an external source of suction not capable to vary its level based on the intrapleural pressure level (i.e., wall suction). The entire definitions and understandings o the abovementioned items, came from the physiology study and research on pleural mechanisms.
The physiology of air leak
The pleural space is a brilliant bioengineering project to accomplish two functions: (I) it maintains the lung perfectly expanded in the chest and (II) it allows a perfect reciprocal sliding of the visceral and parietal pleura with a very low coefficient of friction. All this is accomplished by keeping the pressure of pleural fluid in the sub-atmospheric range that ultimately results from the balance between filtration and drainage of the pleural fluid. Behind the relative simplicity of these concepts there is a well designed and rather complicated system to maintain this situation.
Figure 1 depicts schematically the anatomical arrangement of the pleural space. It is delimited by the visceral pleura wrapping the lung and by the parietal pleura covering the rib cage and diaphragmatic surfaces. Pleural membranes are made of a layer of mesothelial cells, ~4 µm thick, connected to each other by tight junctions on the luminal side and by desmosomes in the subpleural basal part. Mesothelial cells are irregularly covered by microvilli 1-3 µm long varying in density from 2 to 30/µm2. Microvilli trap high concentrations of glycoproteins and hyaluronic acid (15,16). In humans, the thickness of the visceral pleura can attain ~100 µm while that of the parietal pleura is ~5 times less (16-18).The parietal pleura is richly supplied with lymphatics that open directly on the mesothelial surface through the so called lymphatic stomatas as shown in Figure 1A. Stomatas have a diameter ranging from 1 µm up to ~40 µm, have density ranging from 100/cm2 on the intercostal surface up to 8,000/cm2 on the diaphragm (19). The parietal mesothelium of the mediastinal region, is so rich in stomatas to be considered a cribriform membrane (Kampmeier foci). Stomatas are frequently grouped in clusters and connect to an extensive network of submesothelial lacunae (15,20). There is a fractal distribution of lymphatic stomatas on the parietal pleura, a clear indication that their greater density at some places reflects the need for a larger draining capacity (21). The lymphatics of the visceral pleura do not connect directly with the pleural space and therefore, unlike the lymphatics of the parietal pleura, are not involved in the drainage of pleural fluid.
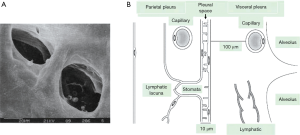
The blood supply to the parietal pleura comes from the systemic circulation, whilst venous drainage is through the intercostal veins (22-24). In the visceral pleura the blood supply originates from the systemic circulation via the bronchial arteries (25) and drains mainly into the pulmonary veins.
Pleural liquid pressure
Let’s model the pleural space as a simple column of water surrounding the lung. In a gravitational field, fluids in static conditions (that is when no flows are present) display an interesting property concerning the gravity dependent distribution of pressures: as shown in Figure 2 left, pressure increases by 1 cmH2O per each cm going down along the water column, this is called “hydrostatic gradient”. Obviously, one can equally think of a decrease in pressure by 1 cmH2O per each cm going up. Let’s take this case (Figure 2 right) simulating the condition of the pleural cavity and imagine to have a system draining pleural fluid at the bottom of the pleural cavity (lymphatics of the diaphragmatic region when standing). Imagine also that the draining system can generate a pressure of about 0 cmH2O (that is equal to atmospheric): it is obvious that pleural liquid pressure should progressively become more subatmospheric going up in the cavity. For a 35-cm height of the pleural cavity, pleural liquid pressure should attain −35 cm at top of the cavity. Direct measurements of pleural liquid pressure in humans are not available but good estimates extrapolating data from large mammals indicate that in supine humans, at mid-heart level, pleural liquid pressure is in the range of −10 cmH2O.
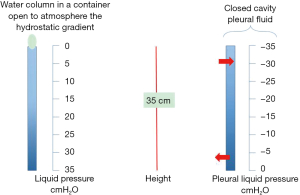
Figure 2 right provides two important informations concerning pleural fluid turnover: fluid drainage mostly occurs at bottom of the cavity through lymphatic drainage, while the rather subatmospheric pressure at top of cavity provides microvascular filtration from the capillaries of the parietal pleura into the pleural space.
We shall now refine this oversimplified schema of Figure 2 right.
The vertical distribution of pleural liquid pressure does in fact not fully reflect a hydrostatic situation. As implicit in Figure 2 right, there ought to be a top to bottom flow of pleural fluid, however this flow is so low as to minimally affect the hydrostatic gradient, although flow resistance within the pleural space is relatively high (26). Furthermore, a flow of pleural fluid was also demonstrated from the costal side to the mediastinal regions that are very rich in lymphatics (27).
Figure 3 is a more realistic model of fluid dynamics in the pleural space showing the polarization of filtration/drainage for pleural fluid. Fluid is produced mostly in the less dependent regions of the parietal pleura. The visceral pleura is essentially excluded from pleural fluid turnover in physiological conditions due to its greater thickness, in fact its permeability is about 10- fold lower compared to that of the parietal pleura (28,29). Finally, pleural fluid is then drained by the lymphatic stomata of the parietal pleura in the diaphragmatic, costal and mediastinal regions (30,31). Flow velocity in initial lymphatics is of the order of 2 mm/min (19). Some data suggest that active water transport operated by mesothelial cells might play some role in pleural fluid turnover (32).
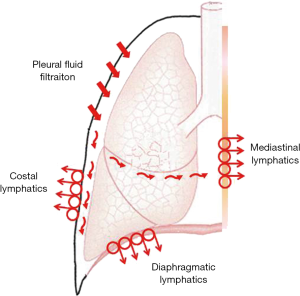
Lubrication
The negative pleural liquid pressure keeps the visceral and the parietal pleura in very close apposition so that the volume of pleural fluid is maintained at the minimum (≈0.3 mL/kg). On physical ground, the visceral and parietal pleura are actually pushing one against the other. Despite this mechanical disposition, no friction of reciprocally sliding pleural membranes is encountered. This occurs because pleural membranes are covered by phospholipids adsorbed and stratified in multiple layers on their surfaces (33). These molecules (Figure 4) have hydrophobic tails carrying negative charges so that the parietal and visceral pleura are not really touching each other due to reciprocal repulsive forces of charges of the same sign (negative) carried by phospholipids. In practice, despite the fact the opposing pleurae are pushing one against the other, they will never touch. In doing so, these molecules provide an efficient lubrication system allowing easy reciprocal sliding of the pleural membranes by keeping the coefficient of friction down to a minimal value (~0.02, as for ice sliding on ice).
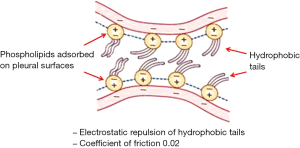
Control of pleural fluid volume
Pleural fluid is hypooncotic (≈1 g/dL protein), proving the low permeability of the parietal pleura to water and proteins. Pleural fluid turnover is estimated to be ≈0.15 mL/kg/h (30), assuring fluid renewal in about 1 h. Lymphatics act as an efficient negative feedback system to regulate pleural fluid dynamics (31) as they can markedly increase the draining flow in response to increased filtration (30,31).
Some features concerning the efficiency of lymphatics to control pleural liquid volume can be briefly summarized (15,31). For a 10-times increase in filtration rate (as in case of inflammation causing an increase in pleural hydraulic permeability), the volume of the liquid would not even be doubled due to the possibility of lymphatics to increase the draining flow. Further, on reducing the maximum lymph draining flow down to 1:10 of normal (e.g., for mediastinal lymphatic compression or mesothelioma) the volume of the liquid would also only increase by 1.5 fold. Yet, with such a strong limitation to lymphatic drainage, any increase in filtration rate could not be met, inevitably resulting in pleural fluid accumulation. These features of the control mechanisms have been developed using data from animal model (15,31); although it appears reasonable to question whether the same mechanism operates in humans it would appear surprising that such a perfect control mechanism would not be present in humans.
Pathophysiology of pleural effusion
On pathophysiological basis, pleural effusion is favoured by: (I) an increase in systemic capillary pressure and an increase in permeability of the parietal/visceral pleura; and (II) a strong limitation to draining lymphatic flow. When pleural effusion develops, the increase in pleural fluid volume will purely reflect a new equilibrium between filtration and drainage. Most cases of pleural effusion simply reflect an inflammatory increase in microvascular permeability. Pleural effusions are classified as transudates or exudates when the fluid/plasma total protein ratio (TPR) is either lower or higher than 0.5 (34-36). An increase in plasma protein is a sign of greater damage to the mesothelium.
Pleural effusion is more frequent in left heart failure (it is present in ~15% of class IV patients (37,38) and could be related to a marked increase in permeability of the visceral pleura. The combined action of hypoxia and inflammatory state can also contribute to the increase in permeability of the visceral pleura. Furthermore, lung edema, by generating positive interstitial pressure in the lung interstitial space, may favour fluid filtration into the pleural cavity.
The increase in right atrial pressure and central venous pressure should also entrain a corresponding increase in filtration rate at capillary level from the parietal pleura, yet, pleural effusion is rarely detected even when right atrial pressure exceeds 20 mmHg (38); the simple interpretation is that the corresponding increase in filtration rate of the parietal pleura can be compensated by lymphatic drainage.
Recovery from pleural effusion
Full recovery from pleural effusion is a long process. Recovery ranges from weeks (for post-myocardial infarction and post-coronary artery by-pass) to months (for tuberculosis and asbestosis) (39). A decrease in pleural fluid volume can only occur when pleural microvascular permeability returns to physiological values reducing pleural filtration to control values.
Mechanical lung chest wall coupling
As noted above, a close apposition of the lung to the chest wall is guaranteed by the subatmospheric pleural liquid pressure. A puzzling question to physiologists has been how to relate the degree of alveolar expansion as a function of height within the chest considering that the lung is exposed to progressively negative pleural liquid pressures. It was tempting to relate alveolar distension at a given height to the corresponding value of pleural liquid pressure. Several studies confirmed indeed that alveoli are indeed more expanded in the apical regions and the degree of expansion decreases down to almost zero at the bottom of the cavity (40-42). However, as shown in Figure 5A, the pleural pressure needed to keep alveoli more expanded at top was only −10 cmH2O and not −35 cmH2O, proving the pleural liquid pressure have nothing to do with local alveolar distension! What is then the origin of the gravity dependent alveolar distension? Figure 5B helps to provide an answer. The lung is thought to be kept hanging within the pleural space by the very negative pleural liquid pressure developing around the apical regions but at the same time is also being deformed by its own weight, as much as a hanging coil (43).
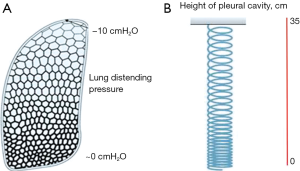
The case of lung resection: identify a strategy for chest drainage
A simple mechanical consideration is that after lung resection a smaller lung will have to fill up a pleural cavity that was previously hosting a larger lung. It is obvious that by removing all air and fluid from the chest will result in overdistension of the alveoli of the resected lung. Good experimental work supports the notion that lung overdistension favors air leak and furthermore represents an edemagenic condition (44).
There are essentially two kinds of strategies for pulmonary re-expansion in the chest after lung resection. On one side, some surgeons, after favoring some air drainage through manual compression of the chest before final closure, only rely on a “passive” strategy of chest drainage. In this case, air and fluid will be progressively cleared from the chest through a fairly long process. Other surgeons adopt the strategy to speed up air and fluid chest drainage by applying “active” suction devices. What is the best, or less hazardous, strategy is still a matter of a stirring debate, considering that post-operative complications are reported to heavily impact on health costs (45).
Along this line, the problem of chest drainage was recently reconsidered by developing the concept of “controlled drainage” as a means to avoid lung overdistension on the hypothesis that this might indeed represent an important co-factor in the development of post-operative complications. A recent article (46) analysed various conditions differing in entity of lung resection (25% and 50% of resected mass) and elastic properties of the lung (normal, fibrotic, emphysematous). The conclusion of the study was that, based on the modified lung-chest wall mechanical coupling after resection, overdistension could be avoided by exposing the operated lung to the same average transpulmonary pressure as before operation. This could be attained by leaving some air and fluid in the chest, based on a relatively simple mechanical index, namely lung compliance measured before and right after lung resection. Along the same line of research (47) an experimental study in animal model demonstrated the individual and combined action of lung resection and hydrothorax on lung compliance. A further study in patients undergoing full lung re-expansion after resection of different entity showed a negative relationship between post-operative lung compliance and total pleural fluid drained. Thus, the study suggested a causative relationship between the decrease in lung compliance and the perturbation in pleuro-pulmonary fluid balance causing hydrothorax.
In summary, the “controlled” drainage may represent a promising option in order to develop an individually tailored chest drainage protocol aiming to manage the complex transition phase towards a new setting of the lung-chest wall mechanical coupling avoiding acute lung overdistension.
Future of air leak research
Scoring systems confirmed, although with different models, that what influences most the parenchyma tearing is probably the underlying chronic disease of the lung.
Future directions of the research in this field are in fact to the basic understandings of the failed or delayed healing of the lung parenchyma. A very recent publication from Bharat and colleagues demonstrated that pleural hypercarbia seems to be associated with persistent alveolo-pleural fistulae following lung resection (48). They analysed intra-pleural gas in 116 patients as elevated CO2 levels have been already reported to inhibit alveolar epithelial proliferation. The practical application of this analysis is related to the assumption of beneficial role of oxygen and suction in the management of the chest tubes.
Future investigations are warranted to investigate the air leak mechanism understanding the pathophysiology underneath the lung resections. With the help of the basic science the surgeon would be able to deliver a patient tailored chest tube protocols and even treatments.
Acknowledgements
Cecilia Pompili has a Research Grant from Medela Healthcare.
Footnote
Conflicts of Interest: The authors have no conflicts of interest to declare.
References
- Varela G, Jiménez MF, Novoa N, et al. Estimating hospital costs attributable to prolonged air leak in pulmonary lobectomy. Eur J Cardiothorac Surg 2005;27:329-33. [PubMed]
- Salati M, Brunelli A, Xiumè F, et al. Does fast-tracking increase the readmission rate after pulmonary resection? A case-matched study. Eur J Cardiothorac Surg 2012;41:1083-7; discussion 1087. [PubMed]
- Pompili C, Salati M, Refai M, et al. Recurrent air leak soon after pulmonary lobectomy: an analysis based on an electronic airflow evaluation†. Eur J Cardiothorac Surg 2015. [Epub ahead of print].
- Varela G, Jiménez MF, Novoa NM, et al. Postoperative chest tube management: measuring air leak using an electronic device decreases variability in the clinical practice. Eur J Cardiothorac Surg 2009;35:28-31. [PubMed]
- Lackey A, Mitchell JD. The cost of air leak: physicians’ and patients’ perspectives. Thorac Surg Clin 2010;20:407-11. [PubMed]
- Pompili C, Tiberi M, Salati M, et al. Patient satisfaction with health-care professionals and structure is not affected by longer hospital stay and complications after lung resection: a case-matched analysis. Interact Cardiovasc Thorac Surg 2015;20:236-41. [PubMed]
- Southey D, Pullinger D, Loggos S, et al. Discharge of thoracic patients on portable digital suction: Is it cost-effective? Asian Cardiovasc Thorac Ann 2015;23:832-8. [PubMed]
- Rieger KM, Wroblewski HA, Brooks JA, et al. Postoperative outpatient chest tube management: initial experience with a new portable system. Ann Thorac Surg 2007;84:630-2. [PubMed]
- Brunelli A, Cassivi SD, Halgren L. Risk factors for prolonged air leak after pulmonary resection. Thorac Surg Clin 2010;20:359-64. [PubMed]
- Brunelli A, Varela G, Refai M, et al. A scoring system to predict the risk of prolonged air leak after lobectomy. Ann Thorac Surg 2010;90:204-9. [PubMed]
- Orsini B, Baste JM, Gossot D, et al. Index of prolonged air leak score validation in case of video-assisted thoracoscopic surgery anatomical lung resection: results of a nationwide study based on the French national thoracic database, EPITHOR†. Eur J Cardiothorac Surg 2015;48:608-11. [PubMed]
- Rivera C, Bernard A, Falcoz PE, et al. Characterization and prediction of prolonged air leak after pulmonary resection: a nationwide study setting up the index of prolonged air leak. Ann Thorac Surg 2011;92:1062-8. [PubMed]
- Brunelli A, Beretta E, Cassivi SD, et al. Consensus definitions to promote an evidence-based approach to management of the pleural space. A collaborative proposal by ESTS, AATS, STS, and GTSC. Eur J Cardiothorac Surg 2011;40:291-7. [PubMed]
- Refai M, Brunelli A, Varela G, et al. The values of intrapleural pressure before the removal of chest tube in non-complicated pulmonary lobectomies. Eur J Cardiothorac Surg 2012;41:831-3. [PubMed]
- Wang NS. Mesothelial cells in situ. In: Chrétien J, Bignon J, Hirsh A, editors. The pleura in health and disease. New York: M. Dekker; 1985:23-42.
- Mariassy AT, Wheeldon EB. The pleura: a combined light microscopic, scanning, and transmission electron microscopic study in the sheep. I. Normal pleura. Exp Lung Res 1983;4:293-314. [PubMed]
- Albertine KH, Wiener-Kronish JP, Staub NC. The structure of the parietal pleura and its relationship to pleural liquid dynamics in sheep. Anat Rec 1984;208:401-9. [PubMed]
- Albertine KH, Wiener-Kronish JP, Roos PJ, et al. Structure, blood supply, and lymphatic vessels of the sheep’s visceral pleura. Am J Anat 1982;165:277-94. [PubMed]
- Miserocchi G, Negrini D. Pleural space: pressures and fluid dynamics. In: Crystal RG, West JB, Weibel ER, et al, editors. The lung: scientific foundations. Vol. 1. New York: Raven Press, 1997:1217-25.
- Negrini D, Del Fabbro M, Gonano C, et al. Distribution of diaphragmatic lymphatic lacunae. J Appl Physiol (1985) 1992;72:1166-72. [PubMed]
- Venturoli D, Grungo S, Negrini D, et al. Fractal Distribution of Serosal Lymphatics. In: Fractals in Biology and Medicine. Springer, 1998:345-53.
- Policard A, Galy P. La plèvre: mécanismes normaux et pathologiques. Paris: Masson, 1942:77-6.
- Rouvière H. Anatomie humaine, descriptive et topographique. T. 2. 9th ed. Paris: Masson, 1962:813-20.
- Leak LV. Gross and ultrastructural morphologic features of the diaphragm. Am Rev Respir Dis 1979;119:3-21. [PubMed]
- Bernaudin JF, Fleury J. Anatomy of the blood and lymphatics circulation of the pleural serosa. In: Chrétien J, Bignon J, Hirsh A, editors. The pleura in health and disease. New York: M. Dekker, 1985:101-24.
- Miserocchi G, Venturoli D, Negrini D, et al. Intrapleural fluid movements described by a porous flow model. J Appl Physiol 1992;73:2511-6. [PubMed]
- Miserocchi G, Nakamura T, Mariani E, et al. Pleural liquid pressure over the interlobar mediastinal and diaphragmatic surfaces of the lung. Respir Physiol 1981;46:61-9. [PubMed]
- Negrini D, Reed RK, Miserocchi G. Permeabiliry-surface area product and reflection coefficient of the parietal pleura in dogs. J Appl Physiol (1985) 1991;71:2543-7.
- Negrini D, Townseley MI, Taylor AE. Hydraulic conductivity and osmotic reflection coefficient of canine parietal pleura in vivo. J Appl Physiol (1985) 1990;69:438-42.
- Miserocchi G. Physiology and pathophysiology of pleural fluid turnover. Eur Respir J 1997;10:219-25. [PubMed]
- Miserocchi G, Venturoli D, Negrini D, et al. Model of pleural fluid turnover. J Appl Physiol (1985) 1993;75:1798-806. [PubMed]
- Zocchi L. Physiology and pathophysiology of pleural fluid turnover. Eur Respir J 2002;20:1545-58. [PubMed]
- Hills BA. Graphite-like lubrication of mesothelium by oligolamellar pleural surfactant. J Appl Physiol (1985) 1992;73:1034-9. [PubMed]
- Joseph J, Badrinath P, Basran GS, et al. Is the pleural fluid transudate or exudate? A revisit of the diagnostic criteria. Thorax 2001;56:867-70. [PubMed]
- Light RW, Macgregor MI, Luchsinger PC, et al. Pleural effusions: the diagnostic separation of transudates and exudates. Ann Intern Med 1972;77:507-13. [PubMed]
- Joseph J, Badrinath P, Basran GS, et al. Do we need all three criteria for the diagnostic separation of pleural fluid into transudates and exudates? An appraisal of the traditional criteria. Med Sci Monit 2003;9:CR474-6. [PubMed]
- Wiener-Kronish JP, Goldstein R, Matthay RA, et al. Lack of association of pleural effusion with chronic pulmonary arterial and right atrial hypertension. Chest 1987;92:967-70. [PubMed]
- Gao ZC, Xue PL, Zhang Y, et al. Potential role of human visceral pleura in pleural liquid turnover. Chin Med J (Engl) 2006;119:250-4. [PubMed]
- Cohen M, Sahn SA. Resolution of pleural effusions. Chest 2001;119:1547-62. [PubMed]
- Glazier JB, Hughes JM, Maloney JE, et al. Vertical gradient of alveolar size in lungs of dogs frozen intact. J Appl Physiol 1967;23:694-705. [PubMed]
- Hoppin FG, Green ID, Mead J. Distribution of pleural surface pressure in dogs. J Appl Physiol 1969;27:863-73. [PubMed]
- Hogg JC, Nepsky S. Regional lung volume and pleural pressure gradient estimated from lung density in dogs. J Appl Physiol 1969;27:198-203. [PubMed]
- West JB, Matthews FL. Stresses, strains, and surface pressures in the lung caused by its weight. J Appl Physiol 1972;32:332-45. [PubMed]
- Miserocchi G, Negrini D, Gonano C. Parenchymal stress affects interstitial and pleural pressures in in situ lung. J Appl Physiol (1985) 1991;71:1967-72. [PubMed]
- Shander A, Fleisher LA, Barie PS, et al. Clinical and economic burden of postoperative pulmonary complications: patient safety summit on definition, risk-reducing interventions, and preventive strategies. Crit Care Med 2011;39:2163-72. [PubMed]
- Miserocchi G, Beretta E, Rivolta I. Respiratory mechanics and fluid dynamics after lung resection surgery. Thorac Surg Clin 2010;20:345-57. [PubMed]
- Salito C, Bovio D, Mazzuca E, et al. Experimental model to evaluate the effect of hydrothorax and lobar resection on lung compliance. Eur J Cardiothorac Surg 2014;45:489-95; discussion 495. [PubMed]
- Bharat A, Graf N, Mullen A, et al. Pleural hypercarbia after lung surgery is associated with persistent alveolopleural fistulae. Chest 2015. [Epub ahead of print]. [PubMed]