Systolic anterior motion of the mitral valve in hypertrophic cardiomyopathy: a narrative review
Introduction
The advent of cardiac ultrasound in the late 1960’s, about a decade after the first descriptions of hypertrophic cardiomyopathy (HCM) and following initial work with angiography, allowed for the site of left ventricular outflow tract (LVOT) obstruction to be identified (1-3). Echocardiographic M-mode examination of 6 HCM patients diagnosed angiographically revealed that the anterior mitral valve leaflet (AML) was apposed to the interventricular septum for approximately 60% of the ejection phase, a phenomenon which has since been termed systolic anterior motion (SAM) of the mitral valve (MV). In that same study, the injection of methoxamine, a systemic vasoconstrictor, led to the disappearance of SAM (4).
Clinically ranging from a silent abnormality diagnosed by cardiac imaging to a dynamic LVOT obstruction with hemodynamic repercussions, SAM has been described in up to 95% of HCM patients (5-7). Approximately 60% to 70% of these patients have either resting or provocable LVOT obstruction (6). In order to fully elucidate the pathophysiology of the SAM-septal contact and LVOT obstruction in HCM, we first present a detailed and timely review on anatomy of the MV apparatus in HCM. Secondly, the various existing theories pertaining to the SAM phenomenon itself as supported by cardiac imaging is examined. Finally, we provide an overview on the medical and interventional management of SAM in HCM. We present the following article in accordance with the Narrative Review reporting checklist (available at https://jtd.amegroups.com/article/view/10.21037/jtd-22-182/rc).
Methods
A literature review was performed using PubMed, EMBASE, Ovid, and the Cochrane Library, of all scientific articles published through December 2021 (Table 1). The Boolean search terms used included: ‘hypertrophic cardiomyopathy’, ‘left ventricular outflow tract obstruction’, ‘systolic anterior motion’, ‘SAM’, ‘mitral valve’, ‘septal myectomy’, ‘septal alcohol ablation’, ‘mitral valve surgery’, ‘mitral valve repair’, ‘mitral valve replacement’, ‘echocardiography’, and ‘cardiac magnetic resonance’. A focus was placed on descriptive studies, reports correlating echocardiographic findings with pathologic diagnosis, and outcomes studies. All authors conducted the literature search, and consensus was reached when three out of four authors agreed on all studies. Additionally, the echocardiography laboratory imaging archives at Columbia University Division of Cardiology, Mount Sinai Heart Institute (Miami Beach, FL, USA) were referenced.
Table 1
Variable | Search details |
---|---|
Date of search | 12/1/2021–12/31/2021 |
Databases searched | PubMed, EMBASE, Ovid, Cochrane Library |
Search items used | ‘hypertrophic cardiomyopathy’, ‘left ventricular outflow tract obstruction’, ‘systolic anterior motion’, ‘SAM’, ‘mitral valve’, ‘septal myectomy’, ‘septal alcohol ablation’, ‘mitral valve surgery’, ‘mitral valve repair’, ‘mitral valve replacement’, ‘echocardiography’, ‘cardiac magnetic resonance’ |
Timeframe of studies | 1/1/1958–12/31/2021 |
Inclusion and exclusion criteria | Focus placed on descriptive studies, reports correlating echocardiographic findings with pathologic diagnosis, and outcomes studies. No exclusion criteria. |
Selection process | All authors conducted the literature search. Consensus was reached by at least two of three authors on all studies |
SAM, systolic anterior motion.
Discussion
Normal mitral valve apparatus anatomy
The normal MV apparatus is a complex structure comprised of the anterior and posterior leaflets, fibromuscular annulus, chordae tendinae, lateral and medial papillary muscles, and subtending left ventricular (LV) myocardium. The valvular tissue attaches circumferentially to the fibromuscular mitral annulus, which is saddle-shaped and dynamic in motion (8,9). The anterior leaflet is longer (18–24 mm) and semicircular in shape, while the posterior leaflet is shorter (11–14 mm) but encompasses a larger surface area. The free edges of the posterior leaflet are indented and create distinct scallops which are named P1 (lateral), P2 (central), and P3 (medial); while no distinct scallops are present on the anterior leaflet, it is customary to divide the tissue visually into counterpart scallops to allow for anatomic description. The chordae tendinae originate from both papillary muscles and serve to prevent leaflet eversion (10). Primary (marginal) chordae insert into the leaflet free edges, superior to which a ridge demarcates the leaflet coaptation zone. Secondary (basal) chordae attach to the anterior leaflet rough zone and posterior leaflet body, while some patients may also display tertiary chordae from the posterobasal LV myocardium directly into the posterior leaflet (11). The two papillary muscles arise from the distal third of the ventricular myocardium and are oriented beneath the correspondingly named valvular commissures (12). The lateral papillary muscle most commonly exhibits a single head, while the medial displays two separate heads. The spatial orientation and dynamic function of the papillary muscles serves to balance the closing-tethering forces on the MV leaflets, and their tensile vectors maintain a posteriorly directed coaptation point away from the LVOT.
Anatomic basis for SAM in HCM
The cardiac anatomy in HCM is characterized by: (I) marked thickening of the interventricular septum; (II) narrowing of the LVOT; and (III) abnormalities of the MV apparatus and papillary muscle orientation (Table 2; Figure 1) (13,14). This results in significant overlap between the LV inflow and outflow tracts resulting in hemodynamic derangements and obstruction. When comparing the MV and ventricular geometry between HCM patients with SAM and normal subjects, it reveals a significantly shorter MV leaflet coaptation-septal distance (C-sept) (12±4 vs. 21±3 mm, P<0.001) and inter-papillary muscle distance (13±5 vs. 18±4, P=0.02), as well as a larger MV tenting volume indexed to body surface area (2.1±1 vs. 0.5±0.3 mL/m2, P<0.001) (15). The MV coaptation point is on average 9 mm from the AML tip versus within 3 mm from the leaflet tip in HCM versus normal patients (16).
Table 2
Mitral valve structure | Common abnormalities |
---|---|
Valvular | Elongated mitral valve leaflets (both anterior and posterior) |
Increased mitral tenting volume | |
Increased distance from coaptation point to anterior leaflet tip (“residual leaflet”) | |
Smaller coaptation-septal distance (C-sept distance) | |
Papillary muscle hypertrophy | |
Papillary muscles | Increased number of papillary muscles |
Displacement and abnormal papillary muscle insertion | |
Shorter interpapillary muscle distance | |
Chordal apparatus | Shortened and fibrotic chordae tendinae |
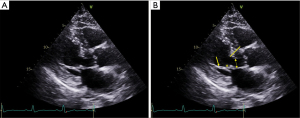
Owing to this unique configuration, the MV in HCM has been termed the “nightcap mitral valve” as it extends into the LV cavity and its coaptation point lies above the plane of the mitral annulus (17). Excess MV leaflet tissue often extends past the coaptation zone and is termed “residual leaflet”, resulting in increased leaflet length and area. This residual MV tissue typically makes the first contact with the septum, and intraventricular hemodynamics in the setting of HCM geometric abnormalities keep the obstructing MV leaflet in the LVOT (18). An examination of 94 MV specimens from HCM patients by Klues and colleagues revealed a significantly increased MV leaflet area compared with controls (12.9±3.7 vs. 8.7±2.0 cm2, P<0.001), mainly driven by increased AML length (Figure 2) (19). The generally accepted normal values for MV leaflet length by echocardiography are 18 to 24 mm for the AML, and 11 to 14 mm for the posterior mitral leaflet (20).
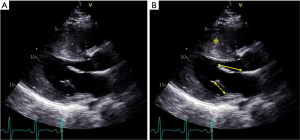
A cardiac magnetic resonance (CMR) study of 172 HCM patients and 15 HCM gene positive/phenotype negative patients detailed the elongation of the MV leaflets (21). The AML length was 26±5 mm and significantly greater than in control subjects (19±5 mm, P<0.001). The length of the posterior mitral leaflet was also significantly greater than that of the controls measuring 14±4 vs. 10±3 mm, respectively (P<0.001). Keeping in mind the differences in measurement techniques between CMR and echocardiography, MV leaflet elongation in HCM patients compared with controls has also been documented by transesophageal echocardiography: 31±4 vs. 22±3 mm for the AML, and 20±2 vs. 15±3 mm for the posterior mitral leaflet (P<0.00001 for both) (16). These structural MV leaflet abnormalities are typically noted in 60% to 70% of HCM patients (19). While a ratio of leaflet length to transverse LVOT diameter >2 has been found to correlate with LVOT obstruction, no relationship has been established between the absolute MV leaflet length and New York Heart Association (NYHA) functional class, severity of mitral regurgitation (MR), maximal LV wall thickness, or the presence of late gadolinium enhancement, so that absolute leaflet length does not seem to be a marker of severity of the disease (22,23).
Papillary muscle hypertrophy, defined as an end-diastolic short-axis thickness of >11 mm on echocardiography, is another anatomical abnormality observed in over 50% of HCM hearts (24,25). CMR characterization of HCM patients has also shown a significant increase in papillary muscle number (2.5 vs. 2.1, P<0.001) and mass as compared with controls (26). In fact, bifid papillary muscles or accessory papillary muscles are frequently identified on imaging (Figure 3). Papillary muscles may also be displaced anteriorly and towards the base, causing an overlap of the LV inflow and outflow, or they may be displaced apically (27). Alternatively, they may fuse with the ventricular septum or to the LV free wall, or may be oriented inwards towards the LV centroid reducing intrapapillary muscle distance and thus creating chordal slack, which in turn may promote SAM. A particularly pathologic variant is insertion of an anomalous papillary muscle directly into the AML causing SAM and LVOT obstruction (28). A type 1 abnormality is characterized by direct insertion of the anomalous papillary muscle into the AML mid-body without chordal attachments; type II has a direct insertion into the AML mid-body with chordal attachments to the leaflet free edge; and, type III has a direct insertion with or without short fibrotic chordae into the AML free edge (29). Papillary muscle abnormalities have been associated with a higher resting LVOT gradient independent of septal thickness or resting heart rate, and are capable of generating SAM in HCM in vitro models with no septal hypertrophy; these abnormalities can be expected in up to 20% of patients (26,27,30).
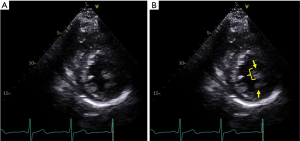
An important subgroup within HCM are the genotype positive-phenotype negative patients, which is also referred to as “pre-clinical” HCM (21,31,32). In these patients LV wall thickness and geometry are commonly normal. However, they are characterized by abnormalities in the MV apparatus anatomy when compared with non-affected individuals, including longer anterior MV leaflets (17.1±0.4 vs. 16±0.4 mm/m2, P=0.006), thicker posterior leaflets (1.79±0.008 vs. 1.62±0.007 cm, P=0.06), and decreased papillary muscle separation (31.1±0.7 vs. 34.2±0.9 mm, P=0.007) (33). Additionally, the prevalence of SAM is also significantly increased in “pre-clinical” HCM (15.2% vs. 1.6%, P=0.006). These abnormalities are more severe in overt genotype and phenotype-positive HCM, which makes a high index of suspicion and comprehensive imaging assessment paramount in identifying these patients for proper risk stratification and treatment.
Mechanical forces responsible for SAM in HCM
Early theories attributed SAM to a Venturi effect whereby narrowing of the LVOT from septal hypertrophy leads to blood flow acceleration due to decreased cross-sectional area, resulting in displacement of the MV leaflets towards the interventricular septum and LVOT via a “suction” force (34). However, timing of SAM has shown that this phenomenon starts even before the onset of systole, when the velocities in the LVOT are still low (35). The technique of vector flow mapping, which combines color Doppler data and speckle tracking analysis, allows for the creation of velocity vectors to characterize the flow of blood inside vascular structures. When applied to HCM hearts, it has shown that in the majority of obstructive HCM, late diastolic mitral inflow creates a posterior vortex pushing the MV leaflets anteriorly, even before the onset of systole (36). This phenomenon has been termed diastolic anterior motion of the MV, which acts as a precursor to SAM. The protruding septum is also responsible for redirecting early, low-velocity systolic flow below to the MV, displacing it anteriorly. It thus appears that in both cases of vortical SAM and ejection SAM, drag forces, as opposed to the Venturi effect, are the main hydraulic forces at play (Figure 4).
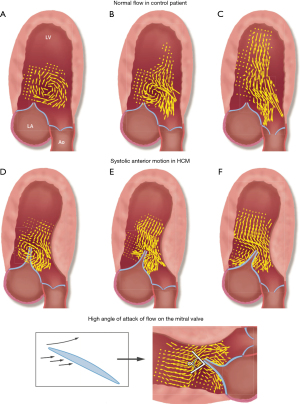
SAM and mitral regurgitation
The degree of MR with SAM is variable, and its severity is correlated with degree of LVOT obstruction, symptomatology, and clinical outcomes (37,38). It typically occurs from poor coaptation of the mitral leaflets which results from the posterior leaflet not being able to move anteriorly with the AML, either because it is too short or because it is not mobile enough (39). Note, however, that primary or degenerative changes of the MV are also common in HCM. These include restrictive or tethered leaflets (70%), de-generative and calcific changes (31%), myxomatous disease (20%), and restrictive chordae (19%) (40). Mitral annular calcification is observed in 19% to 46% of HCM patients with variable degrees of severity (41,42). Annular calcification, particularly when significant posteriorly, decreases the distance between the anterior MV leaflet and coaptation point to the interventricular septum, and displaces the valve apparatus anterior towards the LVOT. When compared to HCM patients without annular calcification, those with the pathology have a greater prevalence of SAM (84% vs. 64%, P<0.001) and LVOT obstruction (81% vs. 58%, P<0.001), and a 46% increased risk of death (P=0.01). Finally, the differentiation between MR secondary to SAM and primary MR is primordial for appropriate management. In order to differentiate the two, the jet direction may be helpful, whereby a posteriorly directed jet usually points to SAM as the etiology of the MR versus a central or anteriorly directed jet which usually indicates primary MV disease (43,44) (Figure 5).
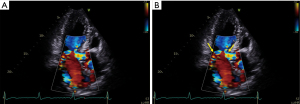
Diagnosis of SAM in HCM
Echocardiography is the modality of choice for diagnosis of HCM and SAM. Care should be taken to evaluate the LV wall thickness, MV leaflet length, and papillary muscle anatomy and orientation (45,46). The maximal interventricular septal and posterior wall thickness is assessed in the parasternal long axis view at end-diastole; maximal apical thickness may be assessed in the parasternal short axis view, or in the apical views, and is enhanced by the use of myocardial contrast. Given their perpendicular orientation to the ultrasound beam, MV leaflet length is also best assessed at mid-diastole in the parasternal long axis orientation. Assessment of the morphology of HCM is also important, as a sigmoid-shaped or reverse curve interventricular septum is more likely to be found in obstructive phenotypes than a neutral hypertrophic septum or apical HCM (47). Color flow Doppler reveals turbulence at the site of SAM-septal contact (Figure 5). SAM severity can be graded as follows: mild for brief SAM without septal contact; moderate for SAM with septal contact lasting <1/3 of the systolic period; and, severe for SAM with septal contact lasting >1/3 of the systolic period (48) (Figure 6). Pulse wave Doppler interrogation at the site of the obstruction should reveal a characteristic “lobster claw” pattern, with a late-peaking signal generally indicating a peak pressure gradient of at least 30 mmHg (49).
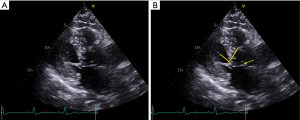
CMR is a critical complementary imaging modality in the detailed assessment of HCM and structural abnormalities of the MV apparatus. It provides superior spatial resolution, tomographic imaging and reconstruction of the heart, and a sharp contrast between the myocardium and blood pool which allows for accurate LV wall thickness measurement and assessment of HCM morphology (50,51). Additionally, CMR has furthered our understanding of HCM-associated papillary muscle abnormalities, and thus provides important insights into the optimal surgical or percutaneous treatments for individual patients (52,53). Limitations to the use of CMR include lack of widespread availability, cost, patient factors such as age, body habitus, and claustrophobia, and certain medical contraindications.
Treatment options
While initial medical management of SAM in HCM includes beta or calcium-channel blockade, volume loading and avoidance of afterload reduction, definitive treatment for refractory symptomatic patients is surgical or percutaneous (45,46). Initially thought to be only present among those with obstructive HCM, SAM has now been described in other entities, which includes post-mitral and aortic valve surgical interventions and hyperdynamic states irrespective of LV hypertrophy (54-56). As previously discussed, anterior displacement of the MV is not only related to dragging forces across the septum but also to abnormalities of the MV apparatus, including increased mitral leaflet area, length, and laxity, as well as anterior displacement of the papillary muscles and chordal abnormalities (7,44,57-60). Therefore, while septal reduction therapy is fundamental in the treatment of LVOT obstruction as it increases the size of the LVOT and the distance to the AML, a global approach considering the MV and papillary muscle geometry should be considered to avoid residual SAM, outflow obstruction or mitral regurgitation (Table 3) (45,46).
Table 3
Technique | Procedure | Mechanism | Results | Caveats |
---|---|---|---|---|
Septal myectomy (Morrow procedure) | Classic: basal IVS resection OR Modified: extended resection to AML-septal contact point | LVOT widening; ↓SAM-generating forces | ↓ LVOT gradients; ↓ SAM by 50–80%; Long-term survival equivalent to general population | VSD; AVB; Persistent LVOTO |
Percutaneous septal alcohol ablation | 96% EtOh injected in 1st septal perforator of LAD | Creation of basal septal myocardial infarction; ↓SAM-generating forces | ↓ LVOT gradients; ↓ SAM by 50% | AVB; Persistent LVOTO; RV infarction |
Resect-plicate-release | (I) ‘Resect’: extended SM; (II) ‘Plicate’: horizontal mid-body AML suture; AND/OR (III) ‘Release’: mobilization of abnormal PM | LVOT widening; ↓ SAM-generating forces; Stiffening of the AML; Posterior positioning of the PM | ↓ LVOT gradients; ↓ SAM by 70–80%; ↓ MR | Caveats of SM; Technical complexity |
Anterior mitral leaflet extension (MLE) | (I) Classic or extended SM; (II) Sewing of a pericardial patch to the central portion of the AML | LVOT widening; ↓ SAM-generating forces; Stiffening of the AML; Posterior displacement of the MV coaptation point | ↓ LVOT gradients; Improved NYHA class symptoms; ↓ Residual SAM; ↓ MR | Caveats of SM; Pericardial patch failure; Recurrent MR; Careful patient selection |
Surgical edge-to-edge repair | (I) Classic or extended SM; (II) Edge-to-edge suture of the A2-P2 MV scallops | LVOT widening; ↓ SAM-generating forces; ↓ MV leaflet motion | ↓ LVOT gradients; Improved NYHA class symptoms; No SAM; No significant MR | Caveats of SM; No use of annuloplasty ring requires careful patient selection; Iaotrogenic MV stenosis |
Anterior mitral leaflet retention plasty | (I) Classic or extended SM; (II) Medial and lateral AML sutured to the posterior annulus | LVOT widening; ↓ SAM-generating forces; Stretches central portion of the AML to prevent SAM | ↓ LVOT gradients; ↑ Functional status; No SAM | Caveats of SM; Small study size; Contraindicated with PM abnormalities |
Secondary chordal cutting | (I) Classic or extended SM; (II) Surgical transection of secondary AML strut chords | LVOT widening; ↓ SAM-generating forces; Relief of AML tethering toward LVOT | ↓ LVOT gradients; ↑ Functional status; ↓ MR | Caveats of SM; Adverse LV remodeling; Careful patient selection |
Transcatheter edge-to-edge mitral valve repair | Transcatheter edge-to-edge repair of the mitral valve in poor surgical candidates | ↓ MV leaflet motion; Anchored MV coaptation point | ↓ LVOT gradients; ↓ MR severity; No SAM | Small case series; Minimal follow-up |
AML, anterior mitral leaflet; AVB, atrioventricular block; EtOh, ethanol; IVS, interventricular septum; LAD, left anterior descending coronary artery; LV, left ventricle; LVOT, left ventricular outflow tract; LVOTO, left ventricular outflow tract obstruction; MLE, mitral leaflet extension; MR, mitral regurgitation; MV, mitral valve; NYHA, New York Heart Association; PM, papillary muscle; RV, right ventricle; SAM, systolic anterior motion; SM, septal myectomy; VSD, ventricular septal defect. ↑: increased; ↓: decreased.
Septal reduction therapy
Septal myectomy (Morrow procedure) is the most widely used and accepted surgical procedure in patients with obstructive HCM resistant to medical therapy. Using a transaortic or transmitral approach, a resection of the basal portion of the interventricular septum is performed, thereby increasing the size of LVOT and eliminating the forces leading to SAM (61). For patients with more extensive septal thickening, the myectomy is extended to the mid-ventricular level distal to the point of AML-septal contact (Modified Morrow procedure) (62). In experienced HCM centers of excellence, myectomy is performed with an operative mortality <1%, effectively reduces septal thickness and LVOT gradient, improves functional status, and is associated with mid-to-long term survival equivalent to that of the general population (63,64).
In patients at high or prohibitive surgical risk, or in preference to surgical intervention, a percutaneous alcohol septal ablation can be performed. The procedure involves injection of small amount of 96% ethanol into the first septal perforator of the left anterior descending coronary artery, effectively causing a basal myocardial infarction and subsequent remodeling of the LVOT (65). When comparing the overall outcomes of septal myectomy and ablation, there is no difference in reported survival; however, patients undergoing myectomy have a greater reduction in LVOT gradient and less need for repeat intervention (66). Detailed registry data have shown that when stratified by 65 years of age, those <65 have superior outcomes with septal myectomy. Patients >65 years old have at least equivalent outcomes with either approach, and thus a less invasive alcohol ablation in this group is reasonable (67).
Of note, SAM may persist in variable degrees of severity post-myectomy in 20 to 50% of patients (68,69). This is influenced by the aforementioned MV apparatus abnormalities inherent to the disease and can result in recurrent symptomatic LVOT obstruction (70). Higher risk features for residual LVOT obstruction include anterior MV leaflet elongation measuring >30 mm, a narrow end-diastolic coaptation point-septal (C-sept) distance measuring <20 mm at end-diastole, moderate to severe SAM, and prominent centrally displaced papillary muscles; these markers identify patients in whom MV intervention with or without myectomy may be considered (17,47,71). The application of MV surgical intervention also becomes particularly useful in patients with less severe septal hypertrophy, defined as a thickness less than 18 mm, in whom standard myectomy increases the risk for creation of a ventricular septal defect (72,73). Finally, choosing a percutaneous approach to treatment of LVOT obstruction does not allow any MV abnormalities to be addressed. Thus, careful assessment of the cause of SAM and LVOT obstruction is of paramount importance to ensure accurate risk stratification and procedure selection.
Resect-plicate-release procedure
The primary component of the ‘Resect-Plicate-Release’ procedure is the extended septal myectomy (‘resect’) (74,75). In patients with an elongated and lax anterior MV leaflet, a horizontal suture is placed at the leaflet mid-body to ‘plicate’, shorten, and stiffen the tissues in order to prevent SAM. For an elongated or anterior displaced anterolateral papillary muscle, ‘release’ of the basal portion of the muscle and abnormal muscular attachments to the anterolateral LV wall allow for its posterior movement away from the LVOT. Halpern and colleagues reported on 77 obstructive HCM patients who underwent myectomy as part of a ‘Resect-Plicate-Release’ procedure; 50 patients underwent AML plication, and 50 underwent anterolateral papillary muscle release (76). At a mean follow-up of 9 months, there was a reduction in LVOT gradient (107 vs. 10 mmHg), MR grade (1.8 vs. 1.2), and presence of SAM (100% vs. 22%), a decrease in AML length in patients undergoing plication, and an increase in the C-sept distance after anterolateral papillary muscle release (P<0.01 for all). Pre-operative echocardiography is used to apply the procedural tenets as follows: (I) ‘Plicate-Release’, with possible limited myectomy, for septal thickness ≤18 mm and AML length >30 mm; (II) ‘Resect-Plicate-Release’ for septal thickness >18 mm and AML length >30 mm; and (III) ‘Resect-Release’ for septal thickness >18 mm and AML ≤30 mm. The surgical expertise required and procedural learning curve are the most limiting aspects of this technique.
Anterior mitral leaflet extension
Introduced in 1991, AML extension consists of sewing a harvested glutaraldehyde-treated pericardial patch to the basal or central portion of the leaflet (77). This area is considered the region where inward bending and protrusion of the leaflet into the LVOT occurs (57,78). By stiffening the base and mid-body of the AML, the technique effectively decreases SAM, reestablishes a posterolateral coaptation point away from the LVOT, and increases chordal tensor forces (79-82). Vriesendorp and colleagues reported on 98 obstructive HCM patients who underwent combined myectomy and AML extension (82). At a mean follow-up of 8 years, there were significant reductions in LVOT gradient (93 vs. 9 mmHg), MR grade (2 vs. 0.5), SAM grade (2.4 vs. 0.1), and New York Heart Association functional class (2.8 vs. 1.3) (P<0.001 for all), with a 15-year cumulative survival rate (83%) comparable to an age and sex-matched cohort (85%) as well as a non-obstructive HCM cohort (83%). In a smaller study of 15 patients treated with myectomy and AML extension, 93% had no recurrent MR, and global longitudinal strain mechanics and LV twist were preserved, at 1-year follow-up (83). Across the two studies 7 (6%) patients required reoperation for recurrent MR, patch failure, or persistent LVOT obstruction. Risk factors for pericardial patch calcification, retraction, and failure include advanced age, chronic kidney disease, abnormal calcium metabolism, and systemic inflammatory disorders (77,84,85). This predisposes to MV repair failure and recurrent MR, and thus, careful patient selection for this technique is advised (77,85,86).
Surgical edge-to-edge repair
The Alfieri technique, or surgical edge-to-edge repair, was introduced in the 1990s as an MV repair option for patients with primary or secondary MR. It is performed by suture approximation of the A2 and P2 scallops of the anterior and posterior leaflets, creating a double-orifice MV and anchoring the coaptation point to prevent SAM (87). Benefits include the ability to perform via a transaortic approach which obviates the need for an atriotomy, a short operative time, and decreased surgical trauma (87-89). In a pooled analysis of 6 studies and 158 HCM patients who underwent septal myectomy and edge-to-edge MV repair, there was a significant reduction in LVOT gradient (82 vs. 16 mmHg), moderate or greater MR (84% vs. 5%), and presence of SAM (96% vs. 0%) at 2.8-year follow-up (P<0.001 for all). Survival at follow-up was 97%, re-operative MV surgery was required in 2%, and 97% were in New York Heart Association Function class I or II (90). Given the lack of an implanted MV annuloplasty ring with this approach, preserved MV annular mechanics and anatomy, and minimal leaflet and annular calcification are important for a durable edge-to-edge repair (89,91).
Anterior mitral leaflet retention plasty
Retention plasty of the AML involves the suturing the medial and lateral AML segments to the fibrous posterior annulus using pericardial pledgets; this prevents displacement of the valvular and subvalvular apparatus anteriorly towards the septum by stretching taut the central portion of the AML (92). Delmo Walter and colleagues performed myectomy combined with AML retention plasty on 57 HCM patients with LVOT obstruction and moderate to severe MR (93). At a long-term follow-up of 17 years, there was a marked reduction in the LVOT gradient (99 vs. 9 mmHg), trivial MR was reported in 87%, and SAM was abolished in all patients (P<0.01 for all). Freedom from reoperation and cumulative survival rate were 92.9% and 91.2%, respectively. The primary selection criteria for AML retention plasty include marked septal thickening, elongated and lax AML, and persistent intraoperative LVOT obstruction or SAM after myectomy. Abnormalities of the papillary muscles, such as direct insertion in the AML, are a contraindication; the technical complexity and learning curve of the technique are also notable.
Secondary chordal cutting
Secondary order chordae of the MV, also known as ‘strut’ chordae, are defined as those which insert beyond the free edge and rough zone of the AML into the mid-body of the leaflet (20). In HCM these chordae may be fibrotic, thickened, or shortened, and in these instances contribute to SAM by tethering the AML anteriorly towards the LVOT. Ferrazzi and colleagues reported on 39 HCM patients who underwent a limited myectomy for mild to moderate septal hypertrophy (septal thickness ≤19 mm) and concomitant secondary chordal surgical transection (‘cutting’) (94). There was no operative mortality, and at a follow-up of 24 months there were significant reductions in LVOT gradient (82 vs. 9 mmHg), moderate or greater MR (56% vs. 16%), and New York Heart Association III or IV symptoms (82% vs. 0%) (P<0.001 for all), with no required reoperations. In a small randomized trial of 48 HCM patients undergoing myectomy comparing additional secondary chordal cutting with edge-to-edge MV repair, late survival was similar (100% vs. 96%, P=0.32), no patients had severe recurrent MR, required reoperation, or had NYHA class III or IV symptoms, and LVOT gradient (17 vs. 20 mmHg, P=0.33) was similar at follow-up (95). The main concern of chordal cutting is the perturbations it may cause in LV systolic and diastolic function, papillary muscle mechanics, ventricular torsion, and global remodeling (96).
MV replacement
Replacement of the MV for relief of LVOT obstruction and SAM is strongly discouraged by HCM experts and societal treatment guidelines, with the exception of co-existent MV disease prohibiting valve repair (i.e., rheumatic heart disease, radiation-induced valvulitis, severe annular calcification, infective endocarditis) (45,46,97). When compared to MV repair, replacement is associated with higher perioperative morbidity, left ventricular remodeling and dysfunction, and a predisposition for prosthesis-related complications (98). In patients undergoing septal myectomy, MV replacement is associated with a 12-fold increase in operative mortality, and a markedly attenuated 10-year survival when compared with patients undergoing MV repair (55.2% vs. 80%, P<0.001) (99,100). An important caveat to note is that the poor outcomes of MV replacement also reflect a generally older and sicker population as compared with those who undergo repair.
Transcatheter edge-to-edge MV repair
Transcatheter edge-to-edge MV repair has become an important treatment option for higher-risk patients with moderate-to-severe primary and secondary MR (101,102). Although not currently approved for patients with HCM, small studies have shown promising evidence. A pooled analysis of 4 studies presented the outcomes of 15 HCM patients who were treated with an edge-to-edge repair using the MitraClip device (Abbott Laboratories, Abbott Park, IL, USA) and were considered poor surgical candidates (103). Treatment resulted in elimination of SAM in all patients, decreased LVOT gradient (75 vs. 11 mmHg), reduction in MR severity, and an improvement in functional capacity. The need for further research in larger groups of patients with long-term follow-up is required before adoption of this technique can be considered.
Summary
The pathophysiology of SAM involves the complex interplay between HCM morphology, MV apparatus anatomic abnormalities, and the labile hemodynamic derangements that characterize each individual patient. SAM is a near universal finding in obstructive HCM patients and its amelioration is a primary goal of medical and interventional therapy. Per the American Heart Association and European Society of Cardiology guidelines recommendations, the initial step in this population is treatment with non-vasodilating beta-blockers, non-dihydropyridine calcium channel blockers, and/or disopyramide (Class I, LOE B-C) (45,46). Mavacamten, a myosin adenosine triphosphatase inhibitor which decreases systolic contraction, stabilizes myosin super-relaxed state, and improves diastolic myocardial relaxation, has also been developed for patients with symptomatic obstructive HCM (104). The EXPLORER-HCM trial of 251 symptomatic HCM patients on maximally-tolerated medical therapy showed that compared with placebo, more patients randomized to Mavacamten achieved a clinical response (30% vs. 17%, P=0.0005) and were in New York Heart Association functional class I (27% vs. 1%, 95% CI: 18.3–34.8%) at 30-week follow-up (105). Nevertheless, Mavacamten is not yet approved for clinical use, and further study is warranted given its side effect profile including LV systolic dysfunction, ventricular tachyarrhythmias, and QTc prolongation.
In patients with refractory obstruction and persistent symptoms, septal reduction therapy should be considered at HCM centers of excellence (Class I, LOE B). In these circumstances surgical septal myectomy is preferred, particularly in younger patients, while percutaneous alcohol septal ablation is a reasonable alternative in higher-risk individuals, advanced age, or patient preference. Finally, MV surgery is generally reserved for patients with mild to moderate septal hypertrophy, intrinsic MV disease, papillary muscle abnormalities, or abnormal leaflet morphology. The choice of reparative technique must be individualized for each patient. It is imperative to note that a class III (LOE B) recommendation is given to the use of MV replacement as the primary treatment for LVOT obstruction.
In conclusion, recent scientific advances in the field of HCM have allowed for a maturation of our understanding of the SAM phenomenon. Cardiac imaging plays a critical role in its diagnosis, treatment, and surveillance, and in our ability to apply the appropriate therapeutic regimens—whether that be medical, surgical, or percutaneous intervention. The increasing prevalence of HCM places an emphasis on continued basic and clinical research to further improve outcomes for this challenging population.
Acknowledgments
Funding: None.
Footnote
Reporting Checklist: The authors have completed the Narrative Review reporting checklist. Available at https://jtd.amegroups.com/article/view/10.21037/jtd-22-182/rc
Conflicts of Interest: All authors have completed the ICMJE uniform disclosure form (available at https://jtd.amegroups.com/article/view/10.21037/jtd-22-182/coif). CGM serves as an unpaid editorial board member of Journal of Thoracic Disease from February 2021 to January 2023. The other authors have no conflicts of interest to declare.
Ethical Statement: The authors are accountable for all aspects of the work in ensuring that questions related to the accuracy or integrity of any part of the work are appropriately investigated and resolved.
Open Access Statement: This is an Open Access article distributed in accordance with the Creative Commons Attribution-NonCommercial-NoDerivs 4.0 International License (CC BY-NC-ND 4.0), which permits the non-commercial replication and distribution of the article with the strict proviso that no changes or edits are made and the original work is properly cited (including links to both the formal publication through the relevant DOI and the license). See: https://creativecommons.org/licenses/by-nc-nd/4.0/.
References
- Teare D. Asymmetrical hypertrophy of the heart in young adults. Br Heart J 1958;20:1-8. [Crossref] [PubMed]
- Morrow AG, Braunwald E. Functional aortic stenosis; a malformation characterized by resistance to left ventricular outflow without anatomic obstruction. Circulation 1959;20:181-9. [Crossref] [PubMed]
- Wigle ED, Heimbecker RO, Gunton RW. Idiopathic ventricular septal hypertrophy causing muscular subaortic stenosis. Circulation 1962;26:325-40. [Crossref] [PubMed]
- Shah PM, Gramiak R, Kramer DH. Ultrasound localization of left ventricular outflow obstruction in hypertrophic obstructive cardiomyopathy. Circulation 1969;40:3-11. [Crossref] [PubMed]
- Maron BJ, Peterson EE, Maron MS, et al. Prevalence of hypertrophic cardiomyopathy in an outpatient population referred for echocardiographic study. Am J Cardiol 1994;73:577-80. [Crossref] [PubMed]
- Ibrahim M, Rao C, Ashrafian H, et al. Modern management of systolic anterior motion of the mitral valve. Eur J Cardiothorac Surg 2012;41:1260-70. [Crossref] [PubMed]
- Pearson AC, Pasierski TJ, Orsinelli DA, et al. Systolic anterior motion of the mitral chordae tendineae: prevalence and clinical and Doppler-echocardiographic features. Am Heart J 1996;131:748-53. [Crossref] [PubMed]
- Levine RA, Handschumacher MD, Sanfilippo AJ, et al. Three-dimensional echocardiographic reconstruction of the mitral valve, with implications for the diagnosis of mitral valve prolapse. Circulation 1989;80:589-98. [Crossref] [PubMed]
- Timek TA, Green GR, Tibayan FA, et al. Aorto-mitral annular dynamics. Ann Thorac Surg 2003;76:1944-50. [Crossref] [PubMed]
- Lam JH, Ranganathan N, Wigle ED, et al. Morphology of the human mitral valve. I. Chordae tendineae: a new classification. Circulation 1970;41:449-58. [Crossref] [PubMed]
- Obadia JF, Casali C, Chassignolle JF, et al. Mitral subvalvular apparatus: different functions of primary and secondary chordae. Circulation 1997;96:3124-8. [Crossref] [PubMed]
- Oosthoek PW, Wenink AC, Wisse LJ, et al. Development of the papillary muscles of the mitral valve: morphogenetic background of parachute-like asymmetric mitral valves and other mitral valve anomalies. J Thorac Cardiovasc Surg 1998;116:36-46. [Crossref] [PubMed]
- Maron BJ, Ommen SR, Semsarian C, et al. Hypertrophic cardiomyopathy: present and future, with translation into contemporary cardiovascular medicine. J Am Coll Cardiol 2014;64:83-99. [Crossref] [PubMed]
- Levine RA, Vlahakes GJ, Lefebvre X, et al. Papillary muscle displacement causes systolic anterior motion of the mitral valve. Experimental validation and insights into the mechanism of subaortic obstruction. Circulation 1995;91:1189-95. [Crossref] [PubMed]
- Hwang HJ, Choi EY, Kwan J, et al. Dynamic change of mitral apparatus as potential cause of left ventricular outflow tract obstruction in hypertrophic cardiomyopathy. Eur J Echocardiogr 2011;12:19-25. [Crossref] [PubMed]
- Grigg LE, Wigle ED, Williams WG, et al. Transesophageal Doppler echocardiography in obstructive hypertrophic cardiomyopathy: clarification of pathophysiology and importance in intraoperative decision making. J Am Coll Cardiol 1992;20:42-52. [Crossref] [PubMed]
- Sherrid MV, Balaram S, Kim B, et al. The Mitral Valve in Obstructive Hypertrophic Cardiomyopathy: A Test in Context. J Am Coll Cardiol 2016;67:1846-58. [Crossref] [PubMed]
- Sherrid MV, Chu CK, Delia E, et al. An echocardiographic study of the fluid mechanics of obstruction in hypertrophic cardiomyopathy. J Am Coll Cardiol 1993;22:816-25. [Crossref] [PubMed]
- Klues HG, Maron BJ, Dollar AL, et al. Diversity of structural mitral valve alterations in hypertrophic cardiomyopathy. Circulation 1992;85:1651-60. [Crossref] [PubMed]
- Dal-Bianco JP, Levine RA. Anatomy of the mitral valve apparatus: role of 2D and 3D echocardiography. Cardiol Clin 2013;31:151-64. [Crossref] [PubMed]
- Maron MS, Olivotto I, Harrigan C, et al. Mitral valve abnormalities identified by cardiovascular magnetic resonance represent a primary phenotypic expression of hypertrophic cardiomyopathy. Circulation 2011;124:40-7. [Crossref] [PubMed]
- Patel P, Dhillon A, Popovic ZB, et al. Left Ventricular Outflow Tract Obstruction in Hypertrophic Cardiomyopathy Patients Without Severe Septal Hypertrophy: Implications of Mitral Valve and Papillary Muscle Abnormalities Assessed Using Cardiac Magnetic Resonance and Echocardiography. Circ Cardiovasc Imaging 2015;8:e003132. [Crossref] [PubMed]
- Lentz Carvalho J, Schaff HV, Nishimura RA, et al. Is anterior mitral valve leaflet length important in outcome of septal myectomy for obstructive hypertrophic cardiomyopathy? J Thorac Cardiovasc Surg 2021; [Epub ahead of print]. [Crossref] [PubMed]
- Silbiger JJ. Abnormalities of the Mitral Apparatus in Hypertrophic Cardiomyopathy: Echocardiographic, Pathophysiologic, and Surgical Insights. J Am Soc Echocardiogr 2016;29:622-39. [Crossref] [PubMed]
- Kobashi A, Suwa M, Ito T, et al. Solitary papillary muscle hypertrophy as a possible form of hypertrophic cardiomyopathy. Jpn Circ J 1998;62:811-6. [Crossref] [PubMed]
- Harrigan CJ, Appelbaum E, Maron BJ, et al. Significance of papillary muscle abnormalities identified by cardiovascular magnetic resonance in hypertrophic cardiomyopathy. Am J Cardiol 2008;101:668-73. [Crossref] [PubMed]
- Kwon DH, Setser RM, Thamilarasan M, et al. Abnormal papillary muscle morphology is independently associated with increased left ventricular outflow tract obstruction in hypertrophic cardiomyopathy. Heart 2008;94:1295-301. [Crossref] [PubMed]
- Klues HG, Schiffers A, Maron BJ. Phenotypic spectrum and patterns of left ventricular hypertrophy in hypertrophic cardiomyopathy: morphologic observations and significance as assessed by two-dimensional echocardiography in 600 patients. J Am Coll Cardiol 1995;26:1699-708. [Crossref] [PubMed]
- Lentz Carvalho J, Schaff HV, Morris CS, et al. Anomalous papillary muscles-Implications in the surgical treatment of hypertrophic obstructive cardiomyopathy. J Thorac Cardiovasc Surg 2022;163:83-89.e1. [Crossref] [PubMed]
- Lefebvre XP, He S, Levine RA, et al. Systolic anterior motion of the mitral valve in hypertrophic cardiomyopathy: an in vitro pulsatile flow study. J Heart Valve Dis 1995;4:422-38. [PubMed]
- Peyrou J, Réant P, Reynaud A, et al. Morphological and functional abnormalities pattern in hypertrophy-free HCM mutation carriers detected with echocardiography. Int J Cardiovasc Imaging 2016;32:1379-89. [Crossref] [PubMed]
- Captur G, Lopes LR, Mohun TJ, et al. Prediction of sarcomere mutations in subclinical hypertrophic cardiomyopathy. Circ Cardiovasc Imaging 2014;7:863-71. [Crossref] [PubMed]
- Groarke JD, Galazka PZ, Cirino AL, et al. Intrinsic mitral valve alterations in hypertrophic cardiomyopathy sarcomere mutation carriers. Eur Heart J Cardiovasc Imaging 2018;19:1109-16. [Crossref] [PubMed]
- Jiang L, Levine RA, King ME, et al. An integrated mechanism for systolic anterior motion of the mitral valve in hypertrophic cardiomyopathy based on echocardiographic observations. Am Heart J 1987;113:633-44. [Crossref] [PubMed]
- Levine RA, Schwammenthal E, Song JK. Diastolic leading to systolic anterior motion: new technology reveals physiology. J Am Coll Cardiol 2014;64:1996-9. [Crossref] [PubMed]
- Ro R, Halpern D, Sahn DJ, et al. Vector flow mapping in obstructive hypertrophic cardiomyopathy to assess the relationship of early systolic left ventricular flow and the mitral valve. J Am Coll Cardiol 2014;64:1984-95. [Crossref] [PubMed]
- Yu EH, Omran AS, Wigle ED, et al. Mitral regurgitation in hypertrophic obstructive cardiomyopathy: relationship to obstruction and relief with myectomy. J Am Coll Cardiol 2000;36:2219-25. [Crossref] [PubMed]
- Feneon D, Schnell F, Galli E, et al. Impact of exercise-induced mitral regurgitation on hypertrophic cardiomyopathy outcomes. Eur Heart J Cardiovasc Imaging 2016;17:1110-7. [Crossref] [PubMed]
- Schwammenthal E, Nakatani S, He S, et al. Mechanism of mitral regurgitation in hypertrophic cardiomyopathy mismatch of posterior to anterior leaflet length and mobility. Circulation 1998;98:856-65. [Crossref] [PubMed]
- Kaple RK, Murphy RT, DiPaola LM, et al. Mitral valve abnormalities in hypertrophic cardiomyopathy: echocardiographic features and surgical outcomes. Ann Thorac Surg 2008;85:1527-35, 1535.e1-2.
- Massera D, Xia Y, Li B, et al. Mitral annular calcification in hypertrophic cardiomyopathy. Int J Cardiol 2022;349:83-9. [Crossref] [PubMed]
- Patlolla SH, Schaff HV, Nishimura RA, et al. Mitral Annular Calcification in Obstructive Hypertrophic Cardiomyopathy: Prevalence and Outcomes. Ann Thorac Surg 2021; [Epub ahead of print]. [Crossref] [PubMed]
- Yeo TC, Miller FA Jr, Oh JK, et al. Hypertrophic cardiomyopathy with obstruction: important diagnostic clue provided by the direction of the mitral regurgitation jet. J Am Soc Echocardiogr 1998;11:61-5. [Crossref] [PubMed]
- Hang D, Schaff HV, Nishimura RA, et al. Accuracy of Jet Direction on Doppler Echocardiography in Identifying the Etiology of Mitral Regurgitation in Obstructive Hypertrophic Cardiomyopathy. J Am Soc Echocardiogr 2019;32:333-40. [Crossref] [PubMed]
- Ommen SR, Mital S, Burke MA, et al. 2020 AHA/ACC Guideline for the Diagnosis and Treatment of Patients With Hypertrophic Cardiomyopathy: A Report of the American College of Cardiology/American Heart Association Joint Committee on Clinical Practice Guidelines. J Am Coll Cardiol 2020;76:e159-240. [Crossref] [PubMed]
- Authors/Task Force members. 2014 ESC Guidelines on diagnosis and management of hypertrophic cardiomyopathy: the Task Force for the Diagnosis and Management of Hypertrophic Cardiomyopathy of the European Society of Cardiology (ESC). Eur Heart J 2014;35:2733-79. [Crossref] [PubMed]
- Rowin EJ, Maron BJ, Maron MS. The Hypertrophic Cardiomyopathy Phenotype Viewed Through the Prism of Multimodality Imaging: Clinical and Etiologic Implications. JACC Cardiovasc Imaging 2020;13:2002-16. [Crossref] [PubMed]
- Pollick C, Rakowski H, Wigle ED. Muscular subaortic stenosis: the quantitative relationship between systolic anterior motion and the pressure gradient. Circulation 1984;69:43-9. [Crossref] [PubMed]
- Sherrid MV, Gunsburg DZ, Pearle G. Mid-systolic drop in left ventricular ejection velocity in obstructive hypertrophic cardiomyopathy--the lobster claw abnormality. J Am Soc Echocardiogr 1997;10:707-12. [Crossref] [PubMed]
- Rickers C, Wilke NM, Jerosch-Herold M, et al. Utility of cardiac magnetic resonance imaging in the diagnosis of hypertrophic cardiomyopathy. Circulation 2005;112:855-61. [Crossref] [PubMed]
- Bois JP, Geske JB, Foley TA, et al. Comparison of Maximal Wall Thickness in Hypertrophic Cardiomyopathy Differs Between Magnetic Resonance Imaging and Transthoracic Echocardiography. Am J Cardiol 2017;119:643-50. [Crossref] [PubMed]
- Maron MS, Maron BJ, Harrigan C, et al. Hypertrophic cardiomyopathy phenotype revisited after 50 years with cardiovascular magnetic resonance. J Am Coll Cardiol 2009;54:220-8. [Crossref] [PubMed]
- To AC, Dhillon A, Desai MY. Cardiac magnetic resonance in hypertrophic cardiomyopathy. JACC Cardiovasc Imaging 2011;4:1123-37. [Crossref] [PubMed]
- Mathew J, Dearani JA, Daly RC, et al. Management of Subaortic Left Ventricular Outflow Tract Obstruction After Aortic Valve Replacement. Ann Thorac Surg 2021;112:1468-73. [Crossref] [PubMed]
- Ashikhmina E, Schaff HV, Daly RC, et al. Risk factors and progression of systolic anterior motion after mitral valve repair. J Thorac Cardiovasc Surg 2021;162:567-77. [Crossref] [PubMed]
- Citro R, Lyon AR, Meimoun P, et al. Standard and advanced echocardiography in takotsubo (stress) cardiomyopathy: clinical and prognostic implications. J Am Soc Echocardiogr 2015;28:57-74. [Crossref] [PubMed]
- Urbano-Moral JA, Gutierrez-Garcia-Moreno L, Rodriguez-Palomares JF, et al. Structural abnormalities in hypertrophic cardiomyopathy beyond left ventricular hypertrophy by multimodality imaging evaluation. Echocardiography 2019;36:1241-52. [Crossref] [PubMed]
- Kim DH, Handschumacher MD, Levine RA, et al. In vivo measurement of mitral leaflet surface area and subvalvular geometry in patients with asymmetrical septal hypertrophy: insights into the mechanism of outflow tract obstruction. Circulation 2010;122:1298-307. [Crossref] [PubMed]
- Maron BJ, Harding AM, Spirito P, et al. Systolic anterior motion of the posterior mitral leaflet: a previously unrecognized cause of dynamic subaortic obstruction in patients with hypertrophic cardiomyopathy. Circulation 1983;68:282-93. [Crossref] [PubMed]
- Moro E, ten Cate FJ, Leonard JJ, et al. Genesis of systolic anterior motion of the mitral valve in hypertrophic cardiomyopathy: an anatomical or dynamic event? Eur Heart J 1987;8:1312-21. [Crossref] [PubMed]
- Morrow AG, Reitz BA, Epstein SE, et al. Operative treatment in hypertrophic subaortic stenosis. Techniques, and the results of pre and postoperative assessments in 83 patients. Circulation 1975;52:88-102. [Crossref] [PubMed]
- Nguyen A, Schaff HV. Transaortic septal myectomy for obstructive hypertrophic cardiomyopathy. Oper Tech Thorac Cardiovasc Surg 2017;22:200-15. [Crossref]
- Wei LM, Thibault DP, Rankin JS, et al. Contemporary Surgical Management of Hypertrophic Cardiomyopathy in the United States. Ann Thorac Surg 2019;107:460-6. [Crossref] [PubMed]
- Nguyen A, Schaff HV, Ommen SR, et al. Late Health Status of Patients Undergoing Myectomy for Obstructive Hypertrophic Cardiomyopathy. Ann Thorac Surg 2021;111:1867-75. [Crossref] [PubMed]
- Sigwart U. Non-surgical myocardial reduction for hypertrophic obstructive cardiomyopathy. Lancet 1995;346:211-4. [Crossref] [PubMed]
- Nguyen A, Schaff HV, Hang D, et al. Surgical myectomy versus alcohol septal ablation for obstructive hypertrophic cardiomyopathy: A propensity score-matched cohort. J Thorac Cardiovasc Surg 2019;157:306-315.e3. [Crossref] [PubMed]
- Sorajja P, Valeti U, Nishimura RA, et al. Outcome of alcohol septal ablation for obstructive hypertrophic cardiomyopathy. Circulation 2008;118:131-9. [Crossref] [PubMed]
- Williams WG, Wigle ED, Rakowski H, et al. Results of surgery for hypertrophic obstructive cardiomyopathy. Circulation 1987;76:V104-8. [PubMed]
- Ralph-Edwards A, Woo A, McCrindle BW, et al. Hypertrophic obstructive cardiomyopathy: comparison of outcomes after myectomy or alcohol ablation adjusted by propensity score. J Thorac Cardiovasc Surg 2005;129:351-8. [Crossref] [PubMed]
- Smedira NG, Lytle BW, Lever HM, et al. Current effectiveness and risks of isolated septal myectomy for hypertrophic obstructive cardiomyopathy. Ann Thorac Surg 2008;85:127-33. [Crossref] [PubMed]
- Zywica K, Jenni R, Pellikka PA, et al. Dynamic left ventricular outflow tract obstruction evoked by exercise echocardiography: prevalence and predictive factors in a prospective study. Eur J Echocardiogr 2008;9:665-71. [Crossref] [PubMed]
- Lapenna E, Nisi T, Carino D, et al. Hypertrophic cardiomyopathy with moderate septal thickness and mitral regurgitation: long-term surgical results. Eur J Cardiothorac Surg 2021;60:244-51. [Crossref] [PubMed]
- Roberts WC. Operative treatment of hypertrophic obstructive cardiomyopathy. The case against mitral valve replacement. Am J Cardiol 1973;32:377-81. [Crossref] [PubMed]
- Schoendube FA, Klues HG, Reith S, et al. Long-term clinical and echocardiographic follow-up after surgical correction of hypertrophic obstructive cardiomyopathy with extended myectomy and reconstruction of the subvalvular mitral apparatus. Circulation 1995;92:II122-7. [Crossref] [PubMed]
- McIntosh CL, Maron BJ, Cannon RO 3rd, et al. Initial results of combined anterior mitral leaflet plication and ventricular septal myotomy-myectomy for relief of left ventricular outflow tract obstruction in patients with hypertrophic cardiomyopathy. Circulation 1992;86:II60-7. [PubMed]
- Halpern DG, Swistel DG, Po JR, et al. Echocardiography before and after resect-plicate-release surgical myectomy for obstructive hypertrophic cardiomyopathy. J Am Soc Echocardiogr 2015;28:1318-28. [Crossref] [PubMed]
- Chauvaud S, Jebara V, Chachques JC, et al. Valve extension with glutaraldehyde-preserved autologous pericardium. Results in mitral valve repair. J Thorac Cardiovasc Surg 1991;102:171-7; discussion 177-8. [Crossref] [PubMed]
- Kofflard MJ, van Herwerden LA, Waldstein DJ, et al. Initial results of combined anterior mitral leaflet extension and myectomy in patients with obstructive hypertrophic cardiomyopathy. J Am Coll Cardiol 1996;28:197-202. [Crossref] [PubMed]
- Lam MT, Wu JC. Biomaterial applications in cardiovascular tissue repair and regeneration. Expert Rev Cardiovasc Ther 2012;10:1039-49. [Crossref] [PubMed]
- van Herwerden LA, Ten Cate FJ. Combined anterior mitral leaflet extension and spark erosion myectomy in hypertrophic obstructive cardiomyopathy: Echo-enhanced surgery. Oper Tech Thorac Cardiovasc Surg 2004;9:310-9. [Crossref]
- van der Lee C, Kofflard MJ, van Herwerden LA, et al. Sustained improvement after combined anterior mitral leaflet extension and myectomy in hypertrophic obstructive cardiomyopathy. Circulation 2003;108:2088-92. [Crossref] [PubMed]
- Vriesendorp PA, Schinkel AF, Soliman OI, et al. Long-term benefit of myectomy and anterior mitral leaflet extension in obstructive hypertrophic cardiomyopathy. Am J Cardiol 2015;115:670-5. [Crossref] [PubMed]
- Fernandez R, Nappi F, Horvath SA, et al. Echocardiographic and clinical outcomes of patients undergoing septal myectomy plus anterior mitral leaflet extension for hypertrophic cardiomyopathy. Rev Cardiovasc Med 2021;22:983-90. [Crossref] [PubMed]
- Mihos CG, Pineda AM, Capoulade R, et al. A Systematic Review of Mitral Valve Repair With Autologous Pericardial Leaflet Augmentation for Rheumatic Mitral Regurgitation. Ann Thorac Surg 2016;102:1400-5. [Crossref] [PubMed]
- Vincentelli A, Zegdi R, Prat A, et al. Mechanical modifications to human pericardium after a brief immersion in 0.625% glutaraldehyde. J Heart Valve Dis 1998;7:24-9. [PubMed]
- Mihos CG, Horvath SA, Fernandez R, et al. Early failure of mitral valve repair with anterior leaflet pericardial patch augmentation in rheumatic and radiation-induced valvulitis. J Thorac Dis 2020;12:2977-82. [Crossref] [PubMed]
- Maisano F, Torracca L, Oppizzi M, et al. The edge-to-edge technique: a simplified method to correct mitral insufficiency. Eur J Cardiothorac Surg 1998;13:240-5; discussion 245-6. [Crossref] [PubMed]
- Källner G, van der Linden J, Hadjinikolaou L, et al. Transaortic approach for the Alfieri stitch. Ann Thorac Surg 2001;71:378-9; discussion 379-80. [Crossref] [PubMed]
- Mihos CG, Larrauri-Reyes M, Hung J, et al. Transaortic Edge-to-Edge Repair for Functional Mitral Regurgitation During Aortic Valve Replacement: A 13-Year Experience. Innovations (Phila) 2016;11:425-9. [Crossref] [PubMed]
- Mihos CG, Escolar E, Fernandez R, et al. A systematic review and pooled analysis of septal myectomy and edge-to-edge mitral valve repair in obstructive hypertrophic cardiomyopathy. Rev Cardiovasc Med 2021;22:1471-7. [Crossref] [PubMed]
- Maisano F, Viganò G, Blasio A, et al. Surgical isolated edge-to-edge mitral valve repair without annuloplasty: clinical proof of the principle for an endovascular approach. EuroIntervention 2006;2:181-6. [PubMed]
- Delmo Walter EM, Siniawski H, Hetzer R. Sustained improvement after combined anterior mitral valve leaflet retention plasty and septal myectomy in preventing systolic anterior motion in hypertrophic obstructive cardiomyopathy in children. Eur J Cardiothorac Surg 2009;36:546-52. [Crossref] [PubMed]
- Delmo Walter EM, Javier MF, Hetzer R. Long-term outcome of simultaneous septal myectomy and anterior mitral leaflet retention plasty in hypertrophic obstructive cardiomyopathy: the Berlin experience. Ann Cardiothorac Surg 2017;6:343-52. [Crossref] [PubMed]
- Ferrazzi P, Spirito P, Iacovoni A, et al. Transaortic Chordal Cutting: Mitral Valve Repair for Obstructive Hypertrophic Cardiomyopathy With Mild Septal Hypertrophy. J Am Coll Cardiol 2015;66:1687-96. [Crossref] [PubMed]
- Afanasyev AV, Bogachev-Prokophiev AV, Zheleznev SI, et al. Edge-to-Edge Repair Versus Secondary Cord Cutting During Septal Myectomy in Patients With Hypertrophic Obstructive Cardiomyopathy: A Pilot Randomised Study. Heart Lung Circ 2021;30:438-45. [Crossref] [PubMed]
- Rodriguez F, Langer F, Harrington KB, et al. Importance of mitral valve second-order chordae for left ventricular geometry, wall thickening mechanics, and global systolic function. Circulation 2004;110:II115-22. [Crossref] [PubMed]
- Afanasyev A, Bogachev-Prokophiev A, Lenko E, et al. Myectomy with mitral valve repair versus replacement in adult patients with hypertrophic obstructive cardiomyopathy: a systematic review and meta-analysis. Interact Cardiovasc Thorac Surg 2019;28:465-72. [Crossref] [PubMed]
- Mick SL, Keshavamurthy S, Gillinov AM. Mitral valve repair versus replacement. Ann Cardiothorac Surg 2015;4:230-7. [PubMed]
- Hong JH, Schaff HV, Nishimura RA, et al. Mitral Regurgitation in Patients With Hypertrophic Obstructive Cardiomyopathy: Implications for Concomitant Valve Procedures. J Am Coll Cardiol 2016;68:1497-504. [Crossref] [PubMed]
- Holst KA, Hanson KT, Ommen SR, et al. Septal Myectomy in Hypertrophic Cardiomyopathy: National Outcomes of Concomitant Mitral Surgery. Mayo Clin Proc 2019;94:66-73. [Crossref] [PubMed]
- Glower DD, Kar S, Trento A, et al. Percutaneous mitral valve repair for mitral regurgitation in high-risk patients: results of the EVEREST II study. J Am Coll Cardiol 2014;64:172-81. [Crossref] [PubMed]
- Stone GW, Lindenfeld J, Abraham WT, et al. Transcatheter mitral-valve repair in patients with heart failure. N Engl J Med 2018;379:2307-18. [Crossref] [PubMed]
- Thomas F, Rader F, Siegel RJ. The use of MitraClip for symptomatic patients with hypertrophic obstructive cardiomyopathy. Cardiology 2017;137:58-61. [Crossref] [PubMed]
- Tower-Rader A, Ramchand J, Nissen SE, et al. Mavacamten: a novel small molecule modulator of β-cardiac myosin for treatment of hypertrophic cardiomyopathy. Expert Opin Investig Drugs 2020;29:1171-8. [Crossref] [PubMed]
- Olivotto I, Oreziak A, Barriales-Villa R, et al. Mavacamten for treatment of symptomatic obstructive hypertrophic cardiomyopathy (EXPLORER-HCM): a randomised, double-blind, placebo-controlled, phase 3 trial. Lancet 2020;396:759-69. [Crossref] [PubMed]