Inflammatory cytokines in robot-assisted thoracic surgery versus video-assisted thoracic surgery
Introduction
Minimally invasive thoracic surgery has been shown to be associated with reduced morbidity, mortality, blood loss, hospital length of stay, and patient pain scores when compared to open thoracotomy (1-3). Recently, robot-assisted thoracic surgery has established its role in the management of patients needing lung resection, and is viewed by many surgeons as the preferred approach to lung resection over video-assisted thoracic surgery (VATS). Each of these approaches has its advantages and disadvantages, in terms of clinical outcomes and cost (4-10). Several early studies have suggested that the VATS approach is associated with lesser release of cytokines and pro-inflammatory mediators, and that may be responsible for many of the benefits of minimally invasive thoracic surgery over open thoracotomy (11-13). It is plausible, that as a minimally invasive approach, the robotic approach would also have lower cytokine and pro-inflammatory mediator release.
However, it is unknown whether the robot-assisted technique is associated with similar or lower pro-inflammatory mediator release compared to VATS. Despite numerous studies comparing the link between perioperative systemic inflammation and morbidity and mortality in VATS versus open thoracotomy, the same comparison has seldom been studied between VATS and the robotic approach (11-13). The more precise dissection afforded by the improved 3-dimensional visualization with the robot may be associated with less tissue trauma and less cytokine release. Similarly, it has been argued that the robotic-assisted thoracic surgery (RATS) approach is associated with a more complete lymphadenectomy and thus potentially reduced oncologic burden, which may not only be associated with a lesser inflammatory state, but also a reduced recurrence rate, however, data regarding this phenomenon is not yet conclusive (4,14-20).
In this context, we conducted a prospective, observational study to assess the overall inflammatory state of patients undergoing VATS or robot-assisted lung resections for suspected or known non-small cell lung cancer (NSCLC) by comparing levels of various interleukins, cytokines, and growth factors. We hypothesized that the robot-assisted approach is associated with less inflammation. We present the following article in accordance with the STROBE reporting checklist (available at https://jtd.amegroups.com/article/view/10.21037/jtd-21-1820/rc).
Methods
Patient population and inclusion criteria
The study was conducted in accordance with the Declaration of Helsinki (as revised in 2013). The study was approved by the Institutional Review Board of Loyola University Medical Center (No. 211663) and informed consent was taken from all individual participants, scheduled to undergo elective lung resection via VATS or robot-assisted techniques. Subjects were recruited between 6/2017–5/2018, and 9/2020–1/2021 at the Loyola University Medical Center, Maywood, IL, USA for this prospective, observational, single-center study. No patients were recruited between 6/2018–9/2020 due to logistical limitations and the coronavirus pandemic. Patients were required to meet the following eligibility criteria: (I) 40+ years old due to a lack of established previous studies detailing the clinic-pathological features and outcomes associated with a younger population; (II) undergoing elective RATS or VATS lung resection for known or suspected NSCLC; and (III) operation to be done by one of two surgeons to reduce operator variability.
Standardized operative techniques, such as incisions and port placements, were implemented for each group as with minimal intragroup operative course heterogeneity. Per convention, CO2 insufflation was used in the robotic approach but not in VATS. To a similar effect, bipolar electrocautery was often used in the robotic cohort but less often in the VATS cohort. The conduct of the operation was similar in each group between patients. Furthermore, all patients underwent a systematic mediastinal and hilar lymph node dissection.
Independent variables
Clinical and demographic data collected included patient age, sex, comorbid conditions, surgical indication, surgical extent, duration of operation, hospital length of stay, and chest tube duration.
Main exposure variable
The surgical approach, VATS or robotic, was the main exposure variable. Surgical approach utilized was based on surgeon preference. Both surgeons were fully proficient in VATS and robotic, thus a learning curve bias was avoided. All operations were performed at one hospital with similar preoperative, intraoperative and postoperative care pathways and protocols.
Blood sample processing
Patient blood samples were collected at three time-points: pre-operatively and 2 and 24 hours following operation completion. Arterial blood samples were drawn directly into sodium citrate tubes and processed immediately via 3000xg centrifugation at +4 ℃ for 30 minutes with obtained plasma aliquoted for preservation at −80 ℃. Immunoassay measurements of interleukin (IL)-1α, IL-1β, IL-2, IL-4, IL-6, IL-8, IL-10, IFN-γ, tumor necrosis factor (TNF)-α, vascular endothelial growth factor (VEGF), monocyte chemo-attractant protein-1 (MCP-1), and endothelial growth factor (EGF) levels were conducted on the Cytokine Biochip Array I using the Evidence Investigator instrumentation from Randox Laboratories (Kearneysville, WV, USA).
Statistical analysis
Clinical and demographic data were collected and analyzed using chi-squared test for categorical variables and two-way analysis of variance for continuous variables as appropriate in order to assess the interaction between both RATS and VATS with lung resection. Bonferroni and Sidak correction were used for multiple comparisons. Data are reported in mean, median, standard deviation (SD), and standard error of the mean (SEM) as appropriate. Patients with missing data were excluded from analysis.
All statistical tests were performed using GraphPad Prism version 8.4.3. All tests were two-sided with alpha set at 0.05.
Results
Patient demographics
This study enrolled 45 patients undergoing minimally invasive anatomic lung resection. Of those, 30 (66.67%) underwent robotic lung resection and 15 (33.33%) underwent VATS anatomic lung resection. Patients were similar in their age, sex, and various other demographic measurements, as shown in Table 1. A statistically significant difference was found in employment status between VATS and robot-assisted, however, this is likely due to the cohorts’ mean ages centering around the US retirement age. No differences were observed in regard to operation, histology, or tumor staging, as shown in Table 2.
Table 1
Variables | VATS, n or mean ± SD | RATS, n or mean ± SD | SE of diff. | 95% CI of diff. | Adjusted P value |
---|---|---|---|---|---|
Sex, male/female | 9/6 | 9/21 | 0.053 | ||
Race | 0.448 | ||||
White | 14 | 24 | |||
Black | 0 | 4 | |||
Asian | 0 | 1 | |||
Hispanic | 1 | 1 | |||
Housing status | 0.382 | ||||
With family/friends | 15 | 25 | |||
Independent | 0 | 4 | |||
Structured/assisted | 0 | 1 | |||
Homeless | 0 | 0 | |||
Employment status | 0.019 | ||||
Retired | 9 | 25 | |||
Employed | 5 | 1 | |||
Unemployed | 1 | 4 | |||
Disabled | 0 | 0 | |||
Age at surgery (years) | 66.4±8.6 | 70±8.6 | 2.16 | −11.70 to 4.51 | >0.99 |
Surgery time (min) | 181.3±56.9 | 168.1±40.1 | 14.28 | −40.46 to 66.85 | >0.99 |
Weight (kg) | 77.38±16.89 | 76.1±17.8 | 4.93 | −17.27 to 19.8 | >0.99 |
Length of stay (days) | 4.1±1.7 | 3.9±3.1 | 0.792 | −2.73 to 3.22 | >0.99 |
POD chest tube removal | 3.2±1.9 | 2.7±3.5 | 0.74 | −2.27 to 3.31 | >0.99 |
PACU medication (morphine eq) | 227.2±230.6 | 204.1±163 | 72.14 | −247.9 to 294.1 | >0.99 |
Post-op day 0 | |||||
Post-op pain day 0 | 3.9±2.2 | 4.6±2 | 0.76 | −3.56 to 2.16 | >0.99 |
Narcotic day 0 (morphine eq) | 16.7±17.5 | 45.4±85 | 23.54 | −117.1 to 59.73 | >0.99 |
Acetaminophen day 0 (mg) | 952.9±681.3 | 1,055±633.4 | 227.3 | −956.2 to 751.2 | >0.99 |
Post-op day 1 | |||||
Post-op pain day 1 | 2.7±1.6 | 3.2±2 | 0.62 | −2.85 to 1.81 | >0.99 |
Narcotic day 1 (morphine eq) | 29.1±38.1 | 33.9±24.8 | 7.6 | −33.70 to 24.13 | >0.99 |
Aspirin day 1 (mg) | 9.5±26.9 | 7.6±24 | 7.4 | −25.85 to 29.72 | >0.99 |
Acetaminophen day 1 (mg) | 1,912±994.3 | 2,082±728.4 | 307.9 | −1,327 to 986.2 | >0.99 |
Post-op day 2 | |||||
Post-op pain day 2 | 2.5±1.5 | 2.6±2.3 | 0.7 | −2.86 to 2.56 | >0.99 |
Narcotic day 2 (morphine eq) | 19.5±15.5 | 18.5±19.2 | 6.31 | −23.36 to 25.42 | >0.99 |
Aspirin day 2 (mg) | 9.5±26.9 | 8.7±25.5 | 8.13 | −30.54 to 32.24 | >0.99 |
Acetaminophen day 2 (mg) | 1,835±1,102 | 1,679±950.7 | 353.7 | −1,210 to 1,522 | >0.99 |
Post-op day 3 | |||||
Post-op pain day 3 | 2.2±1.2 | 2.6±2.6 | 0.89 | −4.36 to 3.50 | >0.99 |
Narcotic day 3 (morphine eq) | 16.1±13.7 | 21.8±24.8 | 7.93 | −40.56 to 29.21 | >0.99 |
Aspirin day 3 (mg) | 10.8±28.5 | 5.4±20.9 | 11.89 | −46.92 to 57.72 | >0.99 |
Acetaminophen day 3 (mg) | 1,517±948.9 | 2,011±994.4 | 397.7 | −2,243 to 1,255 | >0.99 |
Cumulative admission totals | |||||
Narcotic (morphine eq) | 306.6±253.5 | 309.3±234.1 | 86.77 | −328.6 to 323.3 | >0.99 |
Aspirin (mg) | 28.6±80.7 | 17.7±57.2 | 22.12 | −72.23 to 93.97 | >0.99 |
Acetaminophen (mg) | 6,038±3,052 | 5,717±2,639 | 986.1 | −3,383 to 4,025 | >0.99 |
VATS, video-assisted thoracic surgery; RATS, robotic-assisted thoracic surgery; SD, standard deviation; SE, standard error; CI, confidence interval; POD, post-operative day; PACU, post-anesthesia care unit.
Table 2
Variables | VATS, n (%) | RATS, n (%) | Adjusted P value |
---|---|---|---|
Operation | 0.522 | ||
Lobectomy | 7 (46.7) | 15 (50.0) | |
Wedge resection | 3 (20.0) | 4 (13.3) | |
Segmentectomy | 0 | 4 (13.3) | |
Combination | 5 (33.3) | 7 (23.3) | |
Final pathology | 0.558 | ||
Primary | 13 (86.7) | 25 (83.3) | |
Metastasis | 2 (13.3) | 2 (6.7) | |
Other | 0 | 3 (10.0) |
VATS, video-assisted thoracic surgery; RATS, robotic-assisted thoracic surgery.
Plasma cytokine level differences
Two patients from each cohort had missing or incomplete cytokine data, leaving 30 and 15 patients in the robot-assisted and VATS cohorts, respectively.
Pre-operative levels of all cytokines measured were similar between the robotic and VATS cohorts, as shown in Table 3 and Figure 1A.
Table 3
Cytokine | VATS median | RATS median | VATS mean | RATS mean | Mean difference 0 hour | Mean difference 95% CI | Adjusted P value | VATS SEM | RATS SEM |
---|---|---|---|---|---|---|---|---|---|
IL-2 | 0.72 | 0.76 | 0.908 | 0.867 | 0.041 | −15.51 to 15.59 | >0.99 | 0.378 | 0.229 |
IL-4 | 1.56 | 1.51 | 2.421 | 1.513 | 0.907 | −14.64 to 16.46 | >0.99 | 0.865 | 0.102 |
IL-6 | 1.69 | 1.84 | 5.283 | 2.748 | 2.535 | −13.02 to 18.09 | >0.99 | 2.529 | 0.456 |
IL-8 | 4.15 | 4.03 | 9.288 | 4.457 | 4.831 | −10.72 to 20.38 | 0.99 | 3.635 | 0.495 |
IL-10 | 0.49 | 0.46 | 2.476 | 0.617 | 1.859 | −13.69 to 17.41 | >0.99 | 1.916 | 0.122 |
VEGF | 14.79 | 11.69 | 21.26 | 16.58 | 4.673 | −10.88 to 20.22 | 0.99 | 6.001 | 3.172 |
IFNG | 0.27 | 0.31 | 0.242 | 0.723 | −0.481 | −16.03 to 15.07 | >0.99 | 0.042 | 0.291 |
TNFA | 1.51 | 1.46 | 1.501 | 1.652 | −0.151 | −15.70 to 15.40 | >0.99 | 0.217 | 0.227 |
IL-1A | 0.12 | 0.13 | 0.123 | 0.421 | −0.299 | −15.85 to 15.25 | >0.99 | 0.03 | 0.22 |
IL-1B | 1.05 | 1.25 | 1.731 | 9.457 | −7.727 | −23.28 to 7.824 | 0.866 | 0.757 | 5.387 |
MCP-1 | 104.24 | 87.6 | 107.1 | 104.4 | 2.656 | −12.90 to 18.21 | >0.99 | 9.58 | 10.07 |
EGF | 2.61 | 2.65 | 4.044 | 5.653 | −1.61 | −17.16 to 13.94 | >0.99 | 0.887 | 8.602 |
VATS, video-assisted thoracic surgery; RATS, robotic-assisted thoracic surgery; CI, confidence interval; SEM, standard error of the mean; IL, interleukin; VEGF, vascular endothelial growth factor; IFNG, interferon-γ; TNFA, tumor necrosis factor-α; MCP-1, monocyte chemo-attractant protein-1; EGF, endothelial growth factor.
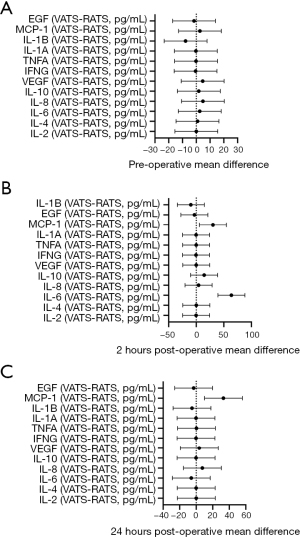
Two-hour post-operative levels of IL-6 and MCP-1 were significantly higher in patients undergoing VATS than in patients undergoing robot-assisted. IL-6 demonstrated the most marked difference between the groups (P=0.001, median =100.88 vs. 41.13 pg/mL, respectively). The difference in MCP-1 levels between VATS and robot-assisted was significant but less robust (P=0.0051, 135.90 vs. 96.90 pg/mL, respectively). Group mean values are shown in Table 4 and Figure 1B (P<0.001 IL-6; P=0.005 MCP-1).
Table 4
Cytokine | VATS median | RATS median | VATS mean |
RATS mean | Mean difference 2 hours | Mean difference 95% CI | Adjusted P value | VATS SEM | RATS SEM |
---|---|---|---|---|---|---|---|---|---|
IL-2 | 0.00 | 0.73 | 0.827 | 0.974 | −0.147 | −24.67 to 24.37 | >0.99 | 0.389 | 0.329 |
IL-4 | 1.68 | 1.50 | 1.638 | 1.526 | 0.113 | −24.41 to 24.63 | >0.99 | 0.083 | 0.077 |
IL-6 | 100.88 | 41.13 | 119 | 55.2 | 63.79 | 39.27 to 88.31 | <0.001 | 16.27 | 10.61 |
IL-8 | 10.25 | 6.70 | 11.76 | 7.492 | 4.272 | −20.25 to 28.79 | >0.99 | 2.951 | 1.074 |
IL-10 | 12.79 | 7.89 | 29.82 | 15.49 | 14.33 | −10.19 to 38.85 | 0.694 | 12.67 | 3.18 |
VEGF | 13.02 | 9.54 | 14.58 | 14.56 | 0.023 | −24.50 to 24.54 | >0.99 | 1.767 | 3.145 |
IFNG | 0.17 | 0.18 | 0.149 | 0.473 | −0.324 | −24.84 to 24.20 | >0.99 | 0.037 | 0.19 |
TNFA | 1.08 | 1.19 | 1.202 | 1.298 | −0.096 | −24.62 to 24.42 | >0.99 | 0.183 | 0.204 |
IL-1A | 0.14 | 0.12 | 0.153 | 0.345 | −0.191 | −24.71 to 24.33 | >0.99 | 0.0423 | 0.197 |
IL-1B | 0.65 | 1.20 | 1.555 | 11.27 | −9.715 | −34.24 to 14.81 | 0.971 | 0.902 | 6.568 |
MCP-1 | 135.93 | 96.90 | 133.8 | 103.5 | 30.28 | 5.763 to 54.80 | 0.005 | 14.41 | 10.37 |
EGF | 0.96 | 1.49 | 0.973 | 4.273 | −3.3 | −27.82 to 21.22 | >0.99 | 0.211 | 1.578 |
VATS, video-assisted thoracic surgery; RATS, robotic-assisted thoracic surgery; CI, confidence interval; SEM, standard error of the mean; IL, interleukin; VEGF, vascular endothelial growth factor; IFNG, interferon-γ; TNFA, tumor necrosis factor-α; MCP-1, monocyte chemo-attractant protein-1; EGF, endothelial growth factor.
Twenty-four-hour post-operative levels of MCP-1 were significantly higher in patients undergoing VATS than in patients undergoing robotic (P=0.001), as shown in Table 5 and Figure 1C.
Table 5
Cytokine | VATS median | RATS median | VATS mean |
RATS mean | Mean difference 24 hours | Mean difference 95% CI | Adjusted P value | VATS SEM | RATS SEM |
---|---|---|---|---|---|---|---|---|---|
IL-2 | 0.84 | 0.81 | 1.029 | 0.905 | 0.124 | −22.78 to 23.03 | 0.358 | 0.162 | 0.046 |
IL-4 | 1.45 | 1.55 | 1.478 | 1.502 | −0.024 | −22.93 to 22.88 | 0.08 | 0.077 | 0.012 |
IL-6 | 44.96 | 38.33 | 39.97 | 45.89 | −5.921 | −28.83 to 16.99 | 7.094 | 6.574 | 1.038 |
IL-8 | 6.64 | 4.52 | 13.220 | 5.724 | 7.498 | −15.41 to 30.40 | 4.732 | 0.683 | 0.577 |
IL-10 | 0.78 | 0.78 | 0.901 | 1.049 | −0.147 | −23.05 to 22.76 | 0.157 | 0.165 | 0.024 |
VEGF | 17.47 | 12.33 | 19.61 | 15.89 | 3.72 | −19.19 to 26.63 | 3.596 | 3.265 | 0.523 |
IFNG | 0.18 | 0.23 | 0.139 | 0.325 | −0.185 | −23.09 to 22.72 | 0.041 | 0.081 | 0.009 |
TNFA | 1.44 | 1.41 | 1.659 | 1.37 | 0.289 | −22.62 to 23.20 | 0.2 | 0.136 | 0.027 |
IL-1A | 0.11 | 0.12 | 0.116 | 0.273 | −0.157 | −23.06 to 22.75 | 0.035 | 0.149 | 0.014 |
IL-1B | 1.00 | 1.81 | 2.83 | 7.894 | −5.064 | −27.97 to 17.84 | 1.826 | 2.53 | 0.315 |
MCP-1 | 164.29 | 131.83 | 169.8 | 136.9 | 32.97 | 10.06 to 55.88 | 18.21 | 14.2 | 2.54 |
EGF | 1.23 | 2.35 | 1.556 | 4.643 | −3.087 | −25.99 to 19.82 | 0.338 | 1.188 | 0.113 |
VATS, video-assisted thoracic surgery; RATS, robotic-assisted thoracic surgery; CI, confidence interval; SEM, standard error of the mean; IL, interleukin; VEGF, vascular endothelial growth factor; IFNG, interferon-γ; TNFA, tumor necrosis factor-α; MCP-1, monocyte chemo-attractant protein-1; EGF, endothelial growth factor.
Clinical outcomes
Assessment of patient demographics, such as population composition and postoperative treatment, showed no statistical difference. The final pathological examination revealed 13 and 25 primary lung cancers in VATS and RATS, respectively, with two patients having undergone a resection for metastasis in each group. Pathology of the remaining three patients in the robotic-assisted group demonstrated inflammatory granulomatous tumors.
Additionally, there was no observed difference in clinical outcomes such as arrhythmias, cardiopulmonary arrests, and prolonged air leaks, as shown in Table 6.
Table 6
Variables | Total (n=49) | VATS (n=17) | RATS (n=32) | P value |
---|---|---|---|---|
Anemia | 1 (2%) | 1 (3%) | >0.99 | |
Arrhythmia | 1 (2%) | 1 (3%) | >0.99 | |
Cardiopulmonary arrest | 1 (2%) | 1 (6%) | 0.354 | |
Hyponatremia | 1 (2%) | 1 (6%) | 0.354 | |
Metabolic encephalopathy | 1 (2%) | 1 (3%) | >0.99 | |
Multifocal ischemic infarct | 1 (2%) | 1 (6%) | 0.333 | |
Pneumothorax | 3 (6%) | 1 (6%) | 2 (6%) | >0.99 |
Pneumomediastinum | 1 (2%) | 1 (3%) | >0.99 | |
Prolonged air leak | 3 (6%) | 2 (12%) | 1 (3%) | 0.273 |
Total | 13 (26%) | 6 (36%) | 7 (22%) | 0.331 |
VATS, video-assisted thoracic surgery; RATS, robotic-assisted thoracic surgery.
Discussion
The implementation of robot-assisted thoracic surgery for the treatment of NSCLC has dramatically increased over the last decade. However, widespread debate regarding its overall efficacy when compared to VATS continues (9,10). In this prospective clinical study, we attempted to address this debate via measurement of blood plasma inflammatory markers in conjunction with patient outcomes in hopes of elucidating the true difference between VATS and RATS in patients undergoing minimally invasive surgery (MIS) lung resection for the treatment of NSCLC. Through the use of sample-matched cytokine measurement data, the current study functions to contribute a linking factor between clinical observations and basic sciences that more homogeneously explores the true variation between the two MIS techniques. Although numerous previous studies have compared VATS to robot-assisted, none were found that included inflammatory biomarker profiling as conducted in the current study (7,14). Additionally, by limiting our study to a single center with no variation in surgeon, we more aptly limited biases and secondary effects as post-matching was free of strong contributors such as MIS learning curves, hospital protocols, surgeon-to-surgeon variability, and surgical team dynamics.
In terms of design and control, our study seemed to have benefited from surgeon consistency as, unlike the majority of previous studies, we had no difference in operation time between VATS and robotic (3,21-25). Additionally, while select previous studies presented statistically significant inter-cohort age differences, we found no difference between our robotic and VATS cohorts (13,26). Thus, we were able to more adequately isolate surgical approach as our exposure variable.
Our results suggest VATS induces a more pronounced inflammatory response than robot-assisted, as demonstrated by measurements of pro-inflammatory cytokines IL-6 and MCP-1 (27,28). These particular findings suggest a greater degree of surgical trauma-induced systemic inflammation as the VATS elevated cytokines have all been strongly associated with thoracic surgery trauma (11-13). Indeed, elevated of pro-inflammatory cytokines, such as IL-6, have been extensively correlated with the degree of cutaneous trauma and subsequently elicited systemic inflammation throughout the repair process (29-32). Thus, the implication of a more pronounced degree of systemic inflammation in the VATS cohort carries a variety of potential clinical manifestations in the peri-operative period such as respiratory failure, renal dysfunction, coagulopathies, and liver dysfunction, however, further studies must be conducted to determine clinical manifestation thresholds (33,34).
Due to prolonged involvement of the immune system in the wound repair processes, our findings may have further time-based implications as the specific elevated inflammatory response observed in VATS patients can lead to dysregulation and reduced efficacy of the immune system (35,36). For example, elevated levels of IL-6, although pro-inflammatory and classically implicated in the production of a robust immune response, can lead to an immunosuppressed state by inhibiting pro-inflammatory IL-1β and TNF-α, both of which play crucial roles in the immuno-competent host response to infection and injury (37,38). Indeed, as TNF-α and IL-1β play pivotal roles in initiating the pro-inflammatory cytokine cascade and mobilizing cells of the immune system, both are rapidly released in substantial quantities following trauma (37,38). Thus, as inducers and predecessors of IL-6 in the cytokine cascade, it would be logical to expect TNF-α and IL-1β levels to rise in conjunction with IL-6 in accordance with the classic cytokine cascade pathway (39,40). However, as depicted data at the 2-hour post-surgery, the lack in statistically significant changes in TNF-α and IL-1β supports the possibility of an IL-6-induced immunosuppressed state and predisposition to infection, as corroborated by previous works (35,39,40). Additional kinetic studies are needed to further validate this notion.
In eliciting elevated MCP-1 levels, VATS also produces potentially negative outcomes as increased MCP-1 levels have been associated with acute kidney injury and increased mortality following thoracic surgery (41). Indeed, in a study of 972 patients, Moledina et al. found MCP-1 levels >196 pg/mL to be associated with significantly increased mortality risk (HR =1.95, 95% CI: 1.09–3.49) (41). In the current study, a significantly larger fraction of VATS patients had MCP-1 levels >196 ng/mL at 24 hours (35.7% vs. 10.3%, P=0.045, χ2=4.013). Similarly, Kremen et al. demonstrated increased MCP-1 levels in the post-operative period following thoracic surgery to be implicated in contributing to post-operative insulin resistance (P<0.05) (42). Hoogeveen et al. demonstrated elevated MCP-1 levels to be an independent factor significantly associated with coronary disease (HR =1.86, 95% CI: 1.36–2.54) (43). Notwithstanding the aforementioned findings, limited population size (N=15) of the Kremen et al. study and inadequate homogeneity amongst the cohort studied by Hoogeveen et al. necessitate more robust studies to more adequately corroborate our findings (42,43).
This study has several limitations. First, this is a single center observational study and is limited by its sample size and imbalanced patient treatment allocation, as such the results may not be generalizable. However, due to the fact that consistent differences were found in cytokine levels despite similar patient cohorts, we believe the results are meaningful. Second, cytokine levels were only measured up to 24 hours post-operation and, as such, we do not have data beyond this timeframe. However, the cytokine levels during first postoperative day are most likely to yield information that pertains to the specific approach used, rather than other postoperative events that may occur which could confound the results. Notwithstanding these limitations, we believe the results are relevant and provide further areas for future studies.
Conclusions
In conclusion, in this single-center, prospective, observational study this study demonstrated that the VATS approach is associated with a more robust pro-inflammatory cytokine response through the upregulation of MCP-1 and IL-6 when compared to the robotic approach in patients undergoing anatomic lung resection. Further studies are necessary in order to determine whether our findings translate to clinically meaningful outcomes.
Acknowledgments
Funding: We would like to graciously thank the Loyola University Chicago, Health Sciences Division, Cardiovascular Research Institute for funding our work.
Footnote
Reporting Checklist: The authors have completed the STROBE reporting checklist. Available at https://jtd.amegroups.com/article/view/10.21037/jtd-21-1820/rc
Data Sharing Statement: Available at https://jtd.amegroups.com/article/view/10.21037/jtd-21-1820/dss
Conflicts of Interest: All authors have completed the ICMJE uniform disclosure form (available at https://jtd.amegroups.com/article/view/10.21037/jtd-21-1820/coif). WJ reports receiving grants from BioFortis, Machaon Diagnostics and KinMaster, consulting fees from Machaon Diagnostics, honoraria from Elsevier, and personal fees from Wilson, Sonsini, Goodrich & Rosati and Lieff, Cabraser, Heimann & Bernstein. The other authors have no conflicts of interest to declare.
Ethical Statement: The authors are accountable for all aspects of the work in ensuring that questions related to the accuracy or integrity of any part of the work are appropriately investigated and resolved. The study was conducted in accordance with the Declaration of Helsinki (as revised in 2013). The study was approved by the Institutional Review Board of Loyola University Medical Center (No. 211663) and informed consent was taken from all individual participants.
Open Access Statement: This is an Open Access article distributed in accordance with the Creative Commons Attribution-NonCommercial-NoDerivs 4.0 International License (CC BY-NC-ND 4.0), which permits the non-commercial replication and distribution of the article with the strict proviso that no changes or edits are made and the original work is properly cited (including links to both the formal publication through the relevant DOI and the license). See: https://creativecommons.org/licenses/by-nc-nd/4.0/.
References
- Louie BE, Wilson JL, Kim S, et al. Comparison of Video-Assisted Thoracoscopic Surgery and Robotic Approaches for Clinical Stage I and Stage II Non-Small Cell Lung Cancer Using The Society of Thoracic Surgeons Database. Ann Thorac Surg 2016;102:917-24. [Crossref] [PubMed]
- Melfi FM, Menconi GF, Mariani AM, et al. Early experience with robotic technology for thoracoscopic surgery. Eur J Cardiothorac Surg 2002;21:864-8. [Crossref] [PubMed]
- Deen SA, Wilson JL, Wilshire CL, et al. Defining the cost of care for lobectomy and segmentectomy: a comparison of open, video-assisted thoracoscopic, and robotic approaches. Ann Thorac Surg 2014;97:1000-7. [Crossref] [PubMed]
- Toker A, Özyurtkan MO, Demirhan Ö, et al. Lymph Node Dissection in Surgery for Lung Cancer: Comparison of Open vs. Video-Assisted vs. Robotic-Assisted Approaches. Ann Thorac Cardiovasc Surg 2016;22:284-90. [Crossref] [PubMed]
- Emmert A, Straube C, Buentzel J, et al. Robotic versus thoracoscopic lung resection: A systematic review and meta-analysis. Medicine (Baltimore) 2017;96:e7633. [Crossref] [PubMed]
- Taniguchi Y, Nakamura H, Miwa K, et al. Initial Results of Robotic Surgery for Primary Lung Cancer: Feasibility, Safety and Learning Curve. Yonago Acta Med 2017;60:162-6. [Crossref] [PubMed]
- Zhou Q, Huang J, Pan F, et al. Operative outcomes and long-term survival of robotic-assisted segmentectomy for stage IA lung cancer compared with video-assisted thoracoscopic segmentectomy. Transl Lung Cancer Res 2020;9:306-15. [Crossref] [PubMed]
- Jaradeh M, Curran B, Vigneswaran WT. Current Status of Minimally Invasive Surgical Approaches for Early Stage Non-Small Cell Lung Cancer: Systematic Review. SCIREA J Clin Med 2020;5:76-88.
- Kent M, Wang T, Whyte R, et al. Open, video-assisted thoracic surgery, and robotic lobectomy: review of a national database. Ann Thorac Surg 2014;97:236-42; discussion 242-4. [Crossref] [PubMed]
- Nishimura JM, Goodwin M, Kneuertz P, et al. Robotic lobectomy costs and quality of life. Mini-Invasive Surg [Internet]. 2020 Feb 14 [cited 2020 Jul 21];2020. Available online: https://misjournal.net/article/view/3340
- Craig SR, Leaver HA, Yap PL, et al. Acute phase responses following minimal access and conventional thoracic surgery. Eur J Cardiothorac Surg 2001;20:455-63. [Crossref] [PubMed]
- Yim AP, Wan S, Lee TW, et al. VATS lobectomy reduces cytokine responses compared with conventional surgery. Ann Thorac Surg 2000;70:243-7. [Crossref] [PubMed]
- Erus S, Öztürk AB, Albayrak Ö, et al. Immune profiling after minimally invasive lobectomy. Interact Cardiovasc Thorac Surg 2021;32:291-7. [Crossref] [PubMed]
- Li C, Hu Y, Huang J, et al. Comparison of robotic-assisted lobectomy with video-assisted thoracic surgery for stage IIB-IIIA non-small cell lung cancer. Transl Lung Cancer Res 2019;8:820-8. [Crossref] [PubMed]
- Kinoshita T, Goto T. Links between Inflammation and Postoperative Cancer Recurrence. J Clin Med 2021;10:228. [Crossref] [PubMed]
- Yang HX, Woo KM, Sima CS, et al. Long-term Survival Based on the Surgical Approach to Lobectomy For Clinical Stage I Nonsmall Cell Lung Cancer: Comparison of Robotic, Video-assisted Thoracic Surgery, and Thoracotomy Lobectomy. Ann Surg 2017;265:431-7. [Crossref] [PubMed]
- Kneuertz PJ, Singer E, D'Souza DM, et al. Hospital cost and clinical effectiveness of robotic-assisted versus video-assisted thoracoscopic and open lobectomy: A propensity score-weighted comparison. J Thorac Cardiovasc Surg 2019;157:2018-2026.e2. [Crossref] [PubMed]
- Ma J, Li X, Zhao S, et al. Robot-assisted thoracic surgery versus video-assisted thoracic surgery for lung lobectomy or segmentectomy in patients with non-small cell lung cancer: a meta-analysis. BMC Cancer 2021;21:498. [Crossref] [PubMed]
- Gallina FT, Melis E, Forcella D, et al. Nodal Upstaging Evaluation After Robotic-Assisted Lobectomy for Early-Stage Non-small Cell Lung Cancer Compared to Video-Assisted Thoracic Surgery and Thoracotomy: A Retrospective Single Center Analysis. Front Surg 2021;8:666158. [Crossref] [PubMed]
- Novellis P, Maisonneuve P, Dieci E, et al. Quality of Life, Postoperative Pain, and Lymph Node Dissection in a Robotic Approach Compared to VATS and OPEN for Early Stage Lung Cancer. J Clin Med 2021;10:1687. [Crossref] [PubMed]
- Lee BE, Korst RJ, Kletsman E, et al. Transitioning from video-assisted thoracic surgical lobectomy to robotics for lung cancer: are there outcomes advantages? J Thorac Cardiovasc Surg 2014;147:724-9. [Crossref] [PubMed]
- Hu X, Wang M. Efficacy and Safety of Robot-assisted Thoracic Surgery (RATS) Compare with Video-assisted Thoracoscopic Surgery (VATS) for Lung Lobectomy in Patients with Non-small Cell Lung Cancer. Comb Chem High Throughput Screen 2019;22:169-78. [Crossref] [PubMed]
- Swanson SJ, Miller DL, McKenna RJ Jr, et al. Comparing robot-assisted thoracic surgical lobectomy with conventional video-assisted thoracic surgical lobectomy and wedge resection: results from a multihospital database (Premier). J Thorac Cardiovasc Surg 2014;147:929-37. [Crossref] [PubMed]
- Augustin F, Bodner J, Maier H, et al. Robotic-assisted minimally invasive vs. thoracoscopic lung lobectomy: comparison of perioperative results in a learning curve setting. Langenbecks Arch Surg 2013;398:895-901. [Crossref] [PubMed]
- Kaur MN, Xie F, Shiwcharan A, et al. Robotic Versus Video-Assisted Thoracoscopic Lung Resection During Early Program Development. Ann Thorac Surg 2018;105:1050-7. [Crossref] [PubMed]
- Jang HJ, Lee HS, Park SY, et al. Comparison of the early robot-assisted lobectomy experience to video-assisted thoracic surgery lobectomy for lung cancer: a single-institution case series matching study. Innovations (Phila) 2011;6:305-10. [Crossref] [PubMed]
- Tanaka T, Narazaki M, Kishimoto T. IL-6 in inflammation, immunity, and disease. Cold Spring Harb Perspect Biol 2014;6:a016295. [Crossref] [PubMed]
- Deshmane SL, Kremlev S, Amini S, et al. Monocyte chemoattractant protein-1 (MCP-1): an overview. J Interferon Cytokine Res 2009;29:313-26. [Crossref] [PubMed]
- Chedid M, Rubin JS, Csaky KG, et al. Regulation of keratinocyte growth factor gene expression by interleukin 1. J Biol Chem 1994;269:10753-7. [Crossref] [PubMed]
- Quayle JA, Adams S, Bucknall RC, et al. Cytokine expression by inflammatory neutrophils. FEMS Immunol Med Microbiol 1994;8:233-9. [Crossref] [PubMed]
- Midwood KS, Williams LV, Schwarzbauer JE. Tissue repair and the dynamics of the extracellular matrix. Int J Biochem Cell Biol 2004;36:1031-7. [Crossref] [PubMed]
- Martin P, Hopkinson-Woolley J, McCluskey J. Growth factors and cutaneous wound repair. Prog Growth Factor Res 1992;4:25-44. [Crossref] [PubMed]
- Edmunds LH Jr. Inflammatory response to cardiopulmonary bypass. Ann Thorac Surg 1998;66:S12-6; discussion S25-8. [Crossref] [PubMed]
- Cremer J, Martin M, Redl H, et al. Systemic inflammatory response syndrome after cardiac operations. Ann Thorac Surg 1996;61:1714-20. [Crossref] [PubMed]
- Dąbrowska AM, Słotwiński R. The immune response to surgery and infection. Cent Eur J Immunol 2014;39:532-7. [Crossref] [PubMed]
- Amodeo G, Bugada D, Franchi S, et al. Immune function after major surgical interventions: the effect of postoperative pain treatment. J Pain Res 2018;11:1297-305. [Crossref] [PubMed]
- Lopez-Castejon G, Brough D. Understanding the mechanism of IL-1β secretion. Cytokine Growth Factor Rev 2011;22:189-95. [Crossref] [PubMed]
- Parameswaran N, Patial S. Tumor necrosis factor-α signaling in macrophages. Crit Rev Eukaryot Gene Expr 2010;20:87-103. [Crossref] [PubMed]
- Tosato G, Jones KD. Interleukin-1 induces interleukin-6 production in peripheral blood monocytes. Blood 1990;75:1305-10. [Crossref] [PubMed]
- Turner NA, Mughal RS, Warburton P, et al. Mechanism of TNFalpha-induced IL-1alpha, IL-1beta and IL-6 expression in human cardiac fibroblasts: effects of statins and thiazolidinediones. Cardiovasc Res 2007;76:81-90. [Crossref] [PubMed]
- Moledina DG, Isguven S, McArthur E, et al. Plasma Monocyte Chemotactic Protein-1 Is Associated With Acute Kidney Injury and Death After Cardiac Operations. Ann Thorac Surg 2017;104:613-20. [Crossref] [PubMed]
- Kremen J, Dolinkova M, Krajickova J, et al. Increased subcutaneous and epicardial adipose tissue production of proinflammatory cytokines in cardiac surgery patients: possible role in postoperative insulin resistance. J Clin Endocrinol Metab 2006;91:4620-7. [Crossref] [PubMed]
- Hoogeveen RC, Morrison A, Boerwinkle E, et al. Plasma MCP-1 level and risk for peripheral arterial disease and incident coronary heart disease: Atherosclerosis Risk in Communities study. Atherosclerosis 2005;183:301-7. [Crossref] [PubMed]