Use of intravascular ultrasound vs. optical coherence tomography for mechanism and patterns of in-stent restenosis among bare metal stents and drug eluting stents
Introduction
In-stent restenosis (ISR) is a histologically distinct pathological process after balloon angioplasty with bare metal stents (BMS), first generation drug-eluting stents (DES), second generation drug-eluting stents (DES), occurring through various mechanisms such as: neointimal hyperplasia, neoatherosclerosis, stent under expansion, and other complications. “Mechanisms and Patterns of Intravascular Ultrasound In-Stent Restenosis Among Bare Metal Stents and First- and Second-Generation Drug-Eluting Stents” by Goto et al., demonstrates the value of intravascular ultrasound (IVUS) in visualizing the mechanisms and patterns of ISR after implanting BMS and first and second generation DES. Although presented through this paper that IVUS is suitable for evaluating and inspecting in stent restenosis, optical coherence tomography (OCT) may provide greater benefit and insight in inspection, and understanding of the mechanisms of ISR.
History of ISR visualization
Coronary angiography has been a gold standard investigation for ISR lesions, especially in providing angiographic classifications for prognostic importance, and therefore can provide appropriate and early patient triage for clinical and investigational purposes (1). Although coronary angiography is commonly used to evaluate ISR lesions, it is limited in its ability to assess ISR in detail. It fails to show differences in the lumen, stent or restenotic tissue area and restenotic tissue. Use of CT coronary angiography allowed reliable detection and quantification of ISR with low radiation exposure (2). Although the results showed high overall diagnostic accuracy, the CT coronary angiography is compromised by several factors; severely calcified arteries, high body mass index, and high heart rates decrease diagnostic accuracy of multidetector CT because of beam-hardening artifacts, excessive image noise, and limited temporal resolution (3). The visualization of the in-stent neointimal hyperplasia with multidetector CT is additionally slowed down by metallic stent struts, because of their high attenuation characteristics and limited spatial resolution of standard-resolution multidetector CT scanner, coronary artery stents are susceptible to partial volume and beam-hardening artifacts. Although decreased collimator width and use of dedicated convolution kernels have been shown to improve stent visualization at multidetector CT, these advances did not overcome artifacts owing to multidetector CT limitations in spatial resolution.
IVUS for ISR
According to the article by Goto et al., IVUS was performed after 0.1−0.2 mg intracoronary nitroglycerine, followed by quantitative IVUS analysis performed using computerized planimetry (4). The IVUS measurements included cross-sectional areas of the external elastic membrane, lumen, stent and NIH. The study was able to confirm the importance of both NIH and chronic stent under expansion as the mechanisms of ISR. The main limitation to the study included inability to evaluate the frequency of neoatherosclerosis because grayscale IVUS is not the technique of choice to assess this phenomenon.
IVUS can be compared to black-and-white TV, where definite imaging is not present therefore does not show as much detail. A situation where IVUS is a suitable choice is a patient with very severely compromised renal function, PCI is planned and aim is trying to minimize contrast usage. Especially if multiple OCT runs are required for vessel sizing or to assess stent expansion then IVUS is a great choice.
OCT for ISR
OCT provides high-definition color images and is a leap forward in assessing coronary vessels from an anatomic standpoint. It has much better resolution, with 10× the axial and lateral resolution of IVUS. OCT has a much faster rotational and pullback speed, and data acquisition only takes 2.5 seconds. This results in obtaining necessary images, and interpreting them with confidence. OCT is easier and faster to set up and use as well. However, OCT requires additional contrast use which is not suitable in case of patients with severe renal dysfunction.
OCT is also superior for guided stent implantation (5). The ILUMIEN system is the first integrated diagnostic technology that combines OCT and FFR in one platform. physiologic and anatomic assessment all in the same system. Reports also show a clear advantage over FD-OCT guided PCI in randomized study (6). OCT provides a 15 um axial resolution, yielding detailed images of his vessel lumen, neointimal tissue and strut distribution (7-12).
OCT patterns of ISR
The high resolution imaging is able to show clear layered appearance of the restenotic tissue, suggesting that the restenosis may be composed of different tissues (13). Pathologic examinations of human atherectomy specimens have demonstrated that restenosis is DES can consist of heterogeneous components including proteoglycan-rich tissue, organized thrombus, atheroma, inflammation and fibrinoid (14). The inner luminal border, the smooth muscles are more compact therefore show a homogeneous concentric orientation, whereas the cell density decreases and appears heterogeneous in the tissue located far from the lumen. Atheromatous material, organized thrombus and inflammatory cells can be observed around the stent struts where the smooth muscle cells are usually oriented in a longitudinal fashion (15). These differences in tissue composition, cell density and orientation comprise in the layered appearance observed. OCT is also successful at identifying structures suggestive of micro vessels in the restenosis, which corresponds to postmortem histology data where the presence of neovascularization in DES restenosis has been described (16,17). The presence of neoatherosclerosis as a cause of late stent failure (18-20) and observation regarding the relationship between lack of stent strut tissue coverage and late/very late stent thrombosis, can be indicted by OCT (21). OCT findings in stent thrombosis may however depend on whether aspiration thrombectomy is performed before or after OCT imaging, due to aspiration’s effect on removing not only thrombus but also fragments of atherosclerotic plaques such as foamy macrophages, cholesterol crystals and thin fibrous cap (22).
IVUS and OCT in comparison
Both systems offer an anatomic assessment of the vasculature and allow visualization into the living, beating hearts. Both of these techniques are used to make measurements for lesion length and lumen size, but OCT is being shown in studies to be more accurate. Intravascular ultrasound (IVUS) is a useful technique to evaluate the extent and distribution of the neointima tissue within the stented segment but is limited to visualize its complex tissue structure as can be documented by histopathology (1,23). Both technologies are analogues as they send out energy waves, OCT uses light and IVUS emits sound waves into the vessel wall and that energy is sent back to the catheter to reconstruct an image, the wavelength of light is much shorter and much faster than sound waves. For this reason, the OCT is able to produce a resolution 10 times greater than IVUS and is able to show much more information. OCT allows us to determine vessel sizing, stent under-expansion, dissection, thrombus, and gives us a good look at intermediate lesions, which on coronary angiography sometimes are hard to determine.
FD-OCT generates similar reference lumen dimensions but higher degrees of disease severity and NIH, as well as better detection of malapposition and tissue prolapsed compared with IVUS (Figure 1). First-generation TD-OCT was associated with smaller reference vessel dimensions compared with IVUS (24). However, an advantage of IVUS is its penetration of 4−8 mm inside the vessel wall. The light-based OCT technology can only penetrate about 2−3 mm. As well as IVUS superior role in contrast limitations and to assess aorto-ostial lesions. The resolution of OCT is far better than IVUS for determining the vessel’s luminal diameter and cross-sectional area. The ability of OCT to provide more detailed visualization of intrastent tissue opens new avenues for tissue characterization and permits establishment of new classification systems for ISR. Moreover, OCT-derived FCT is a good discriminator between ruptured plaque and nonruptured TCFA, while IVUS-derived plaque burden and lumen area had good performance in discriminating RCP from RNCP and TCFA (25). The high-resolution imaging technique is able to evaluate the hyperplastic tissue, demonstrating variation in structure, backscatter and composition (13) that is missed by IVUS in the past (26).
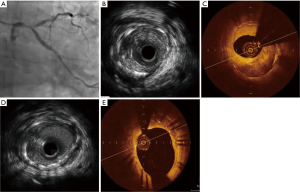
Future of intracoronary imaging
OCT has become a key intracoronary imaging modality capable of overtaking some of the limitations of angiography and intravascular ultrasound. OCT’s imaging with high resolution has given unique insight into not only atheroscclerotic plaque, but also to understanding of tissue responses underlying stent implantation. Further developments with faster OCT pullback speeds will further simplify the procedural requirements and eventually eliminate the need for proximal vessel balloon occlusion during image acquisition. The future developments in OCT technology will see this unique imaging modality become a key player in both the clinical and research arena for the interventional cardiologist. Firstly, an area requiring further exploration, includes widening the clinical indications; for example settings of increased neointima (NI) formation, such as pulmonary hypertension (27), or post-transplantation vasculopathy. Secondly, technological advances currently under investigation are expected to yield improved imaging times and image quality for intravascular OCT. Moreover, the use of various macrophage or other cellular targeting agents labeled with fluorophore, such as annexin A5 and other compounds, may allow better characterization of fibrous cap characteristics using hybrid optical systems (28). However, routine clinical use of OCT will require further clinical trials to validate the technology, establish standard definitions/measurements, and to test its safety and utility in improving clinical outcomes.
Conclusions
Although IVUS is an appropriate choice for assessment of ISR post PCI, IVUS faces certain limitations when comparing the images to OCT; which is able to produce images with higher definition and therefore show more detail. With this evidence, the physician is able to gain a better understanding of the pattern and mechanism of the in-stent restenotic lesions. Moreover, any physician who uses IVUS at the present time has no problem placing the OCT Dragonfly catheter (St. Jude Medical). It’s very small, 2.7 French at the tip, and is very flexible. It is easy to move around curves and significant angulation. Placing the device is not a problem for any interventional cardiologist. Only learning curve is with image interpretation. Interventionalists have to learn how to interpret edge dissection, stent malapposition, vessel sizing, and identify different types of plaque. Although OCT is able to identify differential patterns of restenotic tissue after stenting. This information is helpful in understanding the mechanism of stent restenosis and is useful in further studying of the ISR in the future.
Acknowledgements
None.
Footnote
Provenance: This is an invited article commissioned by the Section Editor Yue Liu (Department of Cardiology, the First Affiliated Hospital of Harbin Medical University, Harbin, China).
Conflicts of Interest: The authors have no conflicts of interest to declare.
References
- Mehran R, Dangas G, Abizaid AS, et al. Angiographic patterns of in-stent restenosis: classification and implications for long-term outcome. Circulation 1999;100:1872-8. [PubMed]
- Andreini D, Pontone G, Mushtaq S, et al. Coronary in-stent restenosis: assessment with CT coronary angiography. Radiology 2012;265:410-7. [PubMed]
- Rixe J, Achenbach S, Ropers D, et al. Assessment of coronary artery stent restenosis by 64-slice multi-detector computed tomography. Eur Heart J 2006;27:2567-72. [PubMed]
- Goto K, Zhao Z, Matsumura M, et al. Mechanisms and Patterns of Intravascular Ultrasound In-Stent Restenosis Among Bare Metal Stents and First- and Second-Generation Drug-Eluting Stents. Am J Cardiol 2015;116:1351-7. [PubMed]
- Waksman R, Kitabata H, Prati F, et al. Intravascular ultrasound versus optical coherence tomography guidance. J Am Coll Cardiol 2013;62:S32-40. [PubMed]
- Habara M, Nasu K, Terashima M, et al. Impact of frequency-domain optical coherence tomography guidance for optimal coronary stent implantation in comparison with intravascular ultrasound guidance. Circ Cardiovasc Interv 2012;5:193-201. [PubMed]
- Byrne RA, Joner M, Tada T, et al. Restenosis in bare metal and drug-eluting stents: distinct mechanistic insights from histopathology and optical intravascular imaging. Minerva Cardioangiol 2012;60:473-89. [PubMed]
- Kimura T, Yokoi H, Nakagawa Y, et al. Three-year follow-up after implantation of metallic coronary-artery stents. N Engl J Med 1996;334:561-6. [PubMed]
- Byrne RA, Iijima R, Mehilli J, et al. Durability of antirestenotic efficacy in drug-eluting stents with and without permanent polymer. JACC Cardiovasc Interv 2009;2:291-9. [PubMed]
- Finn AV, Nakazawa G, Kolodgie FD, et al. Temporal course of neointimal formation after drug-eluting stent placement: is our understanding of restenosis changing? JACC Cardiovasc Interv 2009;2:300-2. [PubMed]
- Fujii K, Mintz GS, Kobayashi Y, et al. Contribution of stent underexpansion to recurrence after sirolimus-eluting stent implantation for in-stent restenosis. Circulation 2004;109:1085-8. [PubMed]
- Alfonso F, Sandoval J, Cárdenas A, et al. Optical coherence tomography: from research to clinical application. Minerva Med 2012;103:441-64. [PubMed]
- Gonzalo N, Serruys PW, Okamura T, et al. Optical coherence tomography patterns of stent restenosis. Am Heart J 2009;158:284-93. [PubMed]
- van Beusekom HM, Saia F, Zindler JD, et al. Drug-eluting stents show delayed healing: paclitaxel more pronounced than sirolimus. Eur Heart J 2007;28:974-9. [PubMed]
- van Beusekom HM, van der Giessen WJ, van Suylen R, et al. Histology after stenting of human saphenous vein bypass grafts: observations from surgically excised grafts 3 to 320 days after stent implantation. J Am Coll Cardiol 1993;21:45-54. [PubMed]
- Schwartz RS, Chronos NA, Virmani R. Preclinical restenosis models and drug-eluting stents: still important, still much to learn. J Am Coll Cardiol 2004;44:1373-85. [PubMed]
- Komatsu R, Ueda M, Naruko T, et al. Neointimal tissue response at sites of coronary stenting in humans: macroscopic, histological, and immunohistochemical analyses. Circulation 1998;98:224-33. [PubMed]
- Takano M, Yamamoto M, Inami S, et al. Appearance of lipid-laden intima and neovascularization after implantation of bare-metal stents extended late-phase observation by intracoronary optical coherence tomography. J Am Coll Cardiol 2009;55:26-32. [PubMed]
- Kang SJ, Mintz GS, Akasaka T, et al. Optical coherence tomographic analysis of in-stent neoatherosclerosis after drug-eluting stent implantation. Circulation 2011;123:2954-63. [PubMed]
- Habara M, Terashima M, Nasu K, et al. Difference of tissue characteristics between early and very late restenosis lesions after bare-metal stent implantation: an optical coherence tomography study. Circ Cardiovasc Interv 2011;4:232-8. [PubMed]
- Mintz GS. Clinical utility of intravascular imaging and physiology in coronary artery disease. J Am Coll Cardiol 2014;64:207-22. [PubMed]
- Yamaji K, Inoue K, Nakahashi T, et al. Bare metal stent thrombosis and in-stent neoatherosclerosis. Circ Cardiovasc Interv 2012;5:47-54. [PubMed]
- Tanimoto S, Aoki J, Serruys PW, et al. Paclitaxel-eluting stent restenosis shows three-layer appearance by optical coherence tomography. EuroIntervention 2006;1:484. [PubMed]
- Bezerra HG, Attizzani GF, Sirbu V, et al. Optical coherence tomography versus intravascular ultrasound to evaluate coronary artery disease and percutaneous coronary intervention. JACC Cardiovasc Interv 2013;6:228-36. [PubMed]
- Tian J, Ren X, Vergallo R, et al. Distinct morphological features of ruptured culprit plaque for acute coronary events compared to those with silent rupture and thin-cap fibroatheroma: a combined optical coherence tomography and intravascular ultrasound study. J Am Coll Cardiol 2014;63:2209-16. [PubMed]
- Saia F, Lemos PA, Sianos G, et al. Effectiveness of sirolimus-eluting stent implantation for recurrent in-stent restenosis after brachytherapy. Am J Cardiol 2003;92:200-3. [PubMed]
- Hou J, Qi H, Zhang M, et al. Pulmonary vascular changes in pulmonary hypertension: optical coherence tomography findings. Circ Cardiovasc Imaging 2010;3:344-5. [PubMed]
- Tahara N, Imaizumi T, Virmani R, et al. Clinical feasibility of molecular imaging of plaque inflammation in atherosclerosis. J Nucl Med 2009;50:331-4. [PubMed]