Non-syndromal mitral valve prolapse (MVP): a common entity, but not commonly associated with DCHS1 or FLNA mutations
Mitral valve prolapse (MVP) is a common echocardiographic finding in the general population (2–3%) and is characterized by morphological and functional abnormalities of the mitral leaflets such as valve insufficiency or mitral annulus disjunction (1). From a clinical point of view, isolated cardiac (or non-syndromal) forms (nMVP) and syndromal forms (with extra-cardiac manifestations; sMVP) can be distinguished, e.g., in the setting of genetic connective tissue disorders such as Marfan, Loeys-Dietz or Ehlers-Danlos syndrome (1). Non-syndromal MVP can be subdivided in three major subphenotypes: myxomatous MVP, fibroelastic deficiency (FED), and Filamin A (FLNA)-MVP (1). The term ‘mitral valve prolapse syndrome’ is used when ventricular arrhythmia occur in the setting of myocardial or papillary fibrosis around the mitral valve. To date, sMVP is caused by >10 genes. In addition, genome-wide association studies reported several loci associated with mitral valve disease (2-6).
In our study, we focused on sporadic cases with nMVP to address the variant frequency in two reported genes [Dachsous cadherin-related protein 1 (DCHS1) and Filamin A (FLNA)]. Mutations in the DCHS1 gene are involved in calcium-dependent cell-cell adhesion (7-9). DCHS1 acts as part of the planar cell polarity (PCP) signaling pathway that controls cytoskeleton rearrangements, cell migration, and plays an important role in morphogenesis (1). Very recently, molecular interactions between DCHS1, cytoplasmic proteins Lix1-Like (LIX1L) and septin-9 (SEPT9) (DCHS1-LIX1L-SEPT9 protein complex) were identified. This complex interacts with the actin cytoskeleton and promotes polymerization of filamentous SEPT9. Disturbance of DCHS1-LIX1L-SEPT9 complex results in disruption of the actin cytoskeleton, cell-tissue organization, and valvulogenesis (10). So far, in Human Gene Mutation Database (HGMD®) (http://www.hgmd.cf.ac.uk) only 30 DCHS1 variants are listed, responsible for different phenotypes. Out of these, 22 are listed as ‘questionable disease causing’, whereas 8 were ‘disease causing’. Two of these variants are directly associated with nMVP. Other phenotypic associations were sudden infant death syndrome or sudden unexplained death, which might be associated with nMVP.
Together with functional data of Dchs1+/− and Dchs1−/− mice (showing abnormal mitral valve formation) and knockdown of Dchs1 by morpholino injection in zebrafish (showing abnormal atrioventricular development and absence of the atrioventricular constriction) the gene has a strong pathogenic evidence and disease validity (7).
In addition to DCHS1, mutations in the X-chromosomal filamin A (FLNA) gene, encoding a cytoskeletal actin-binding protein, known to cause a myxomatous form of valvular dystrophy (11) with a more severe phenotype in male than in female patients with a polyvalvular involvement, mitral leaflet thickening and elongation. Apart from a few FLNA mutations in nMVP, the majority of FLNA mutations are related to connective tissue disorders with skeletal, cardiovascular, and/or gastrointestinal manifestations. Filamin A is known to interact with >70 proteins to regulate key cell properties such as proliferation, differentiation, migration, and extracellular matrix (ECM) densification. The pathophysiologic mechanisms how FLNA mutations may lead to nMVP are not known exactly, but a disturbed mechanotransduction and organization of the filamentous actin network together with impaired signaling pathways are likely (1). Recently, a FLNA knock-in rat model showed that inflammation, epithelial cell migration, or mechanical transduction pathways activated in these mitral valves might help to understand the pathophysiological aspects of nMVP (12).
Since nMVP is associated with various genetic pathways and causes a high rate of sudden unexplained deaths (>10%) or sudden cardiac death (SCD) event rate (0.14/100 patient-years in a MVP cohort) (13), we addressed the frequency of DCHS1 and FLNA gene mutations in an in-house cohort of 40 unrelated patients (34 female vs. 6 male; mean age: 41±14 years) with nMVP. Out of these, only 3 (7.5%) had a positive family history for MVP and 21 (52.5%) had a complicated, rhythmogenic course (either by ventricular arrhythmia, survived SCD or isolated ventricular premature beats; ‘mitral valve prolapse syndrome’) and 4 (10%) with QTc prolongation. All coding exons were directly sequenced via the Sanger method for rare variants in DCHS1 (NM_003737.3) and FLNA (NM_001110556.1).
Overall, in the FLNA gene in our cohort no mutations were detected indicating a minor role for nMVP. In DCHS1, two novel non-synonymous, heterozygous variants were detected, p.Ala2479Thr (c.7435G>A) and p.Ile1069Lys (c.3206T>A). The bioinformatic analysis tool VarCards (http://varcards.biols.ac.cn) which uses a combination of more than 60 genomic and bioinformatic data sources for a comprehensive in-silico assessment of the potential pathogenicity was applied, but showed only a low strength for pathogenicity (6/23 and 2/23 judged as pathogenic; ACMG class 3 [p.Ala2479Thr: PP1, PM1, PM2; p.Ile1069Lys: PP1, PM2), variant of uncertain significance]. However, this has also been noted for the four previously reported causal variants (range: 3/23 till 16/23; Figure 1A), which may reflect that these tools still may not be sufficient enough in this setting. All variants are listed as very rare (<0.05%) in genome Aggregation Database (gnomAD) (Figure 1A). In case of p.Ala2479Thr, these variant is located near other known DCHS1 mutations which are held responsible for MVP (Figure 1B). The DCHS1 gene has a z-score of 2.4 and a pLI-score of 1, which indicates increased constraint (intolerance to variation) and also loss of function events. So the variants found in this study could have an impact on the development of nMVP, although the majority of predictive programs indicate that this is not the case.
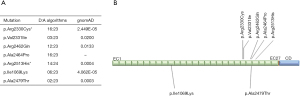
In conclusion, the genetic or ontogenetic pathogenesis of nMVP is largely unclear. Two established disease-causing genes FLNA and DCHS1 do not play a dominant role in the mainly sporadic affected patient collective examined here. Further functional analyses are necessary to show a correlation between the newly identified DCHS1 variants and the development of nMVP.
Acknowledgments
Funding: None.
Footnote
Provenance and Peer Review: This article was a standard submission to the journal. The article has undergone external peer review.
Conflicts of Interest: All authors have completed the ICMJE uniform disclosure form (available at https://jtd.amegroups.com/article/view/10.21037/jtd-22-173/coif). The authors have no conflicts of interest to declare.
Ethical Statement: The authors are accountable for all aspects of the work in ensuring that questions related to the accuracy or integrity of any part of the work are appropriately investigated and resolved. The study was conducted in accordance with the Declaration of Helsinki (as revised in 2013). The study was approved by institutional ethics board of University Hospital Muenster, Muenster, Germany 3IIBahr [AI0910sch] and informed consent was taken from all the patients.
Open Access Statement: This is an Open Access article distributed in accordance with the Creative Commons Attribution-NonCommercial-NoDerivs 4.0 International License (CC BY-NC-ND 4.0), which permits the non-commercial replication and distribution of the article with the strict proviso that no changes or edits are made and the original work is properly cited (including links to both the formal publication through the relevant DOI and the license). See: https://creativecommons.org/licenses/by-nc-nd/4.0/.
References
- Le Tourneau T, Merot J, Rimbert A, et al. Genetics of syndromic and non-syndromic mitral valve prolapse. Heart 2018;104:978-84. [Crossref] [PubMed]
- Dina C, Bouatia-Naji N, Tucker N, et al. Genetic association analyses highlight biological pathways underlying mitral valve prolapse. Nat Genet 2015;47:1206-11. [Crossref] [PubMed]
- Yu M, Georges A, Tucker NR, et al. Genome-Wide Association Study-Driven Gene-Set Analyses, Genetic, and Functional Follow-Up Suggest GLIS1 as a Susceptibility Gene for Mitral Valve Prolapse. Circ Genom Precis Med 2019;12:e002497. [Crossref] [PubMed]
- Yu M, Kyryachenko S, Debette S, et al. Genome-Wide Association Meta-Analysis Supports Genes Involved in Valve and Cardiac Development to Associate With Mitral Valve Prolapse. Circ Genom Precis Med 2021;14:e003148. [Crossref] [PubMed]
- Roselli C, Yu M, Nauffal V, et al. Genome-wide association study reveals novel genetic loci: a new polygenic risk score for mitral valve prolapse. Eur Heart J 2022;43:1668-80. [Crossref] [PubMed]
- Guicciardi NA, De Bonis M, Di Resta C, et al. Genetic background of mitral valve prolapse. Rev Cardiovasc Med 2022;23:96. [Crossref] [PubMed]
- Durst R, Sauls K, Peal DS, et al. Mutations in DCHS1 cause mitral valve prolapse. Nature 2015;525:109-13. [Crossref] [PubMed]
- Boudoulas KD, Pitsis AA, Theofilogiannakos EK, et al. Floppy Mitral Valve/Mitral Valve Prolapse (FMV/MVP): An unrevealed genotype - Phenotype relationship. Hellenic J Cardiol 2020;61:354-6. [Crossref] [PubMed]
- Clemenceau A, Berube JC, Belanger P, et al. Deleterious variants in DCHS1 are prevalent in sporadic cases of mitral valve prolapse. Mol Genet Genomic Med 2018;6:114-20. [Crossref] [PubMed]
- Moore KS, Moore R, Fulmer DB, et al. DCHS1, Lix1L, and the Septin Cytoskeleton: Molecular and Developmental Etiology of Mitral Valve Prolapse. J Cardiovasc Dev Dis 2022;9:62. [Crossref] [PubMed]
- Kyndt F, Gueffet JP, Probst V, et al. Mutations in the gene encoding filamin A as a cause for familial cardiac valvular dystrophy. Circulation 2007;115:40-9. [Crossref] [PubMed]
- Delwarde C, Aumond P, Toquet C, et al. Functional, structural and molecular characterization of a new mitral valve prolapse animal model: The FLNA-P637Q KI rat. Available online:
10.1093/eurheartj/ehab724.1559 10.1093/eurheartj/ehab724.1559 - Nalliah CJ, Mahajan R, Elliott AD, et al. Mitral valve prolapse and sudden cardiac death: a systematic review and meta-analysis. Heart 2019;105:144-51. [Crossref] [PubMed]