To explore the prognostic value of spread through air spaces and develop a nomogram combined with spread through air spaces in lung squamous cell carcinoma
Introduction
GLOBOCAN 2020 (1) showed that although female breast cancer has surpassed lung cancer as the most common cancer worldwide, lung cancer is still the leading cause of cancer death. Despite many new advances in treatment, the prognosis of non-small cell lung cancer (NSCLC) remains poor. Schegoleva et al. (2) demonstrated that even if NSCLC is diagnosed at stage I and II, nearly 1/4 of patients will have postoperative recurrence. Recently, new immunophenotypes or molecular markers have emerged in NSCLC that have related prognostic and predictive functions, including previously underestimated morphological parameters which are considered to affect prognosis. These include improved staging criteria (3) as well as tumor distance to the resection margin, tumor budding, single-cell invasion around the tumor, and spread through air spaces (STAS). In 2015, STAS was identified as a new invasive pattern in the classification of lung tumors proposed by the World Health Organization (WHO). The definition (4) is as follows: “STAS consists of micropapillary clusters, solid nests, or single cells beyond the edge of the tumor into air spaces in the surrounding lung parenchyma”. Moreover, STAS is not included in the percentage measurement of subtype patterns in comprehensive histological typing or in the measurement of invasive size. It is an invasion pattern similar to pleural invasion and vascular invasion. In the updated classification of thoracic tumors in 2021 (5), the WHO reiterated that as a histological feature, STAS has important prognostic significance. Since STAS can be accurately observed in pathological sections, it is controversial whether sublobectomy should be performed as a treatment for small lung cancers (less than 2 cm in diameter). R0 resection did not completely remove part of the alveolar cavity of STAS. On conventional hematoxylin-eosin (H&E)-stained sections, clusters of small or large tumor cells were lined by STAS in the alveoli surrounding the main tumor. Many retrospective studies have shown that STAS is closely related to the prognosis of lung cancer (6-10). According to some studies published in the past 2 years, the incidence of STAS in NSCLC is 15.7% to 48.1% (7-9,11-16). In a prognostic model of invasive lung adenocarcinoma based on pathological features (17), STAS is a significant prognostic factor for regressionfree survival (RFS) and OS. However, TNM stage and related clinical features were not included in the model. STAS was initially recognized in lung adenocarcinoma, and subsequent studies in lung adenocarcinoma have emerged one after another, while there are few studies on STAS in lung squamous cell carcinoma. Therefore, in this study, we determined the pathological features and survival of 540 patients with lung squamous cell carcinoma to explore the value of STAS in predicting the prognosis of postoperative patients with lung squamous cell carcinoma. And we sought to investigate whether a prognostic model including STAS combined with clinical characteristics could significantly predict PFS in lung squamous cell carcinomas. We hope that by adding the factor of STAS, the prediction accuracy of prognosis can be improved. We present the following article in accordance with the TRIPOD reporting checklist (available at https://jtd.amegroups.com/article/view/10.21037/jtd-22-1065/rc).
Methods
Patients and samples
From January 2011 to December 2015, a total of 2302 patients underwent lung cancer surgery in The Affiliated Lihuili Hospital, Ningbo University. Under the inclusion criteria: (I) initial diagnosis is lung squamous cell carcinoma; (II) no intraoperative metastasis was observed; (III) no induction therapy; (IV) patients diagnosed the disease as a solitary disease; (V) negative surgical margin; (VI) the follow-up data is relatively complete. A total of 540 patients were enrolled in this retrospective study. The study was conducted in accordance with the Declaration of Helsinki (as revised in 2013). The study was approved by the ethics committee of Ningbo Medical Center Lihuili Hospital (The Affiliated Lihuili Hospital, Ningbo University) (No. KY2019PJ058) and individual consent for this retrospective analysis was waived. The clinical staging of patients was determined according to the 8th edition of the American Joint Committee on Cancer (AJCC) TNM staging criteria. STAS was re-evaluated by pathologists at Ningbo Clinicopathological Diagnosis Center. Progression-free survival (PFS) referred to the time from randomization to the first occurrence of disease progression or death of any cause. Recurrences were confirmed by clinical, radiological or pathological assessment. We obtained the survival information (including survival or death, tumor recurrence) of patients by telephone follow-up and outpatient follow-up. For the first 3 years, follow-up was conducted every 6 months. After 3 years, patients with stage I were followed up every year, and patients with stage II and III were followed up every 6 months. PFS referred to the time from randomization to the first occurrence of disease progression or death of any cause. The assessment of recurrence was confirmed by clinical, radiological or pathological information. In this study, patients were randomly divided into the discovery cohort (n=324) and validation cohort (n=216) according to the proportion of 6:4.
Statistical analysis
We carried out the t-test for continuous variables and chi-square test for categorical variables to compare differences between the two groups. The survival time of STAS-positive/negative patients was estimated by Kaplan-Meier survival analysis and the log-rank test. We used receiver operating characteristic (ROC) curves to study the predictive performance of STAS. The ‘pROC’ package was applied to perform the ROC curve analysis. Univariate and multivariate Cox regression analysis were applied to analyze the independent prognostic effect of STAS. In multivariate analysis, AJCC stage, tumor differentiation and STAS were independent predictors of PFS. Cox regression coefficients were used to construct a nomogram for predicting the probability of PFS. Calibration plots were derived based on the regression analysis. We assessed the clinical utility of the nomogram by decision curve analysis (DCA). The nomogram and calibration plots were generated using the ‘rms’ R package. Statistical analysis was performed with R software (version 3.0.1) and statistical levels were two-sided. Statistical significance was set at 0.05.
Results
Clinical characteristics of patients
All patients in the cohort (540 patients) underwent surgical resection, and 196 (36.3%) patients were reported to be STAS positive. The clinical staging of patients was determined according to the 8th edition of the AJCC TNM staging criteria. The clinical information of the patients were obtained from medical records and pathological reports. Table 1 presents information on the discovery cohort (n=324) and validation cohort (n=216).
Table 1
Variables | Discovery cohort (n=324) | Validation cohort (n=216) | |||
---|---|---|---|---|---|
STAS (−) | STAS (+) | STAS (−) | STAS (+) | ||
Gender | |||||
Male | 196 | 110 | 127 | 77 | |
Female | 14 | 4 | 7 | 5 | |
Age | |||||
≥60 years | 138 | 77 | 91 | 52 | |
<60 years | 72 | 37 | 43 | 30 | |
Smoker | |||||
No | 79 | 33 | 43 | 25 | |
Former + current | 131 | 81 | 91 | 57 | |
Differentiation | |||||
Low | 69 | 53 | 55 | 32 | |
Moderately | 122 | 49 | 59 | 38 | |
Well | 19 | 12 | 20 | 12 | |
Invasion* | |||||
Vessel (+) | 27 | 36 | 18 | 19 | |
Neural (+) | 27 | 19 | 13 | 5 | |
Pleural (+) | 23 | 29 | 12 | 11 | |
TNM stage | |||||
I | 115 | 28 | 76 | 25 | |
II | 53 | 37 | 34 | 18 | |
III | 42 | 49 | 24 | 39 |
*, the negative data are not included. STAS, spread through air spaces; TNM, tumor, node, metastasis.
Evaluation of the status of STAS
According to the definition of STAS given by the WHO in the classification of lung tumors in 2021: “STAS consists of micropapillary clusters, solid nests, or single cells beyond the edge of the tumor into air spaces in the surrounding lung parenchyma” (5). STAS status was confirmed in all 540 patients. STAS mainly presents as 3 morphological patterns (18): (I) micropapillary structures consisting of papillary structures without central fibrovascular cores which occasionally form ring-like structures within air spaces; (II) solid nests or tumor islands consisting of solid collections of tumor cells filling air spaces; and (III) single cells consisting of scattered discohesive single cells. The artifacts identified with STAS mentioned in related research (18,19) mainly focus on the following categories: (I) mechanically induced tumor floaters are often randomly located at the edges or planes of tissue sections; (II) alveolar macrophages; (III) jagged edges of tumor cell clusters, suggesting tumor fragmentation or edges cut by a knife during specimen handling; (IV) linear tumor cells detached from alveolar walls; (V) benign epithelial cells, such as hyperplastic alveolar parietal cells or bronchial epithelial cells; (VI) solitary tumor clusters distant from the tumor, not spreading continuously from the edge of the tumor.
The correlation between STAS and PFS
There was a significant difference in survival time among patients who were grouped according to their STAS status. The 324 patients in the discovery cohort were further divided into a STAS-negative group and STAS-positive group. Patients who were STAS negative typically had longer 5-year PFS than patients who were STAS positive (P<0.001; Figure 1A). The same analysis was performed in the validation cohort (n=216). According to the pathological results, these patients were also divided into a STAS-negative group and STAS-positive group. The 5-year PFS in the STAS-negative group was also better than that in the STAS-positive group (P<0.001; Figure 1B). In the comprehensive analysis of 540 patients according to negative/positive STAS status, there was still a similar difference in 5-year PFS between the two groups (P<0.001; Figure 1C).
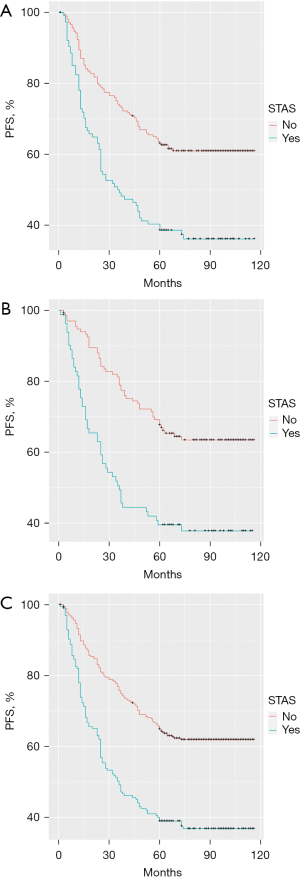
Prediction accuracy of STAS
In the univariate analysis, smoking status, vascular invasion, neural invasion, pleural invasion, AJCC stage, degree of differentiation, and STAS were significant prognostic factors, while there was no significant difference in other clinicopathological factors (Table 2). Multivariate analysis showed that AJCC stage, differentiation, and STAS were still independent predictors of PFS (Table 2). We used ROC curve analysis to evaluate the predictive performance of each model. The area under the curve (AUC) is measured on a scale of 0.5 (no better than chance) to 1 (perfect discrimination). A good prognostic prediction model will have an AUC value closer to 1. The results showed that the AUC of the STAS model was 0.612, the AUC of the AJCC staging model was 0.693, the AUC of the combined STAS and AJCC staging model was 0.713, and the AUC of the combined STAS, AJCC staging, and tumor differentiation status model was 0.720 (Figure 2). The graph intuitively showed that although STAS had a certain diagnostic value, the value of AJCC staging was better than STAS. The AUC of the combination of STAS and AJCC staging was higher than that of AJCC staging alone (using the DeLong’s test method, P=0.018). Therefore, we believe that when AJCC staging is combined with STAS, higher predictive performance can be obtained, that is, the prognostic value of AJCC staging in predicting the risk of recurrence and survival is increased. Also, we used ROC curve analysis to evaluate the predictive performance of model 5 in validation cohort with the AUC of 0.693 (Figure 2).
Table 2
Variables | Univariate | Multivariate | |||
---|---|---|---|---|---|
P value | P value | HR | 95% CI | ||
Age | 0.818 | – | – | – | |
Smoker | 0.0118 | 0.054 | 1.426 | 0.994–2.046 | |
Gender | 0.643 | – | – | – | |
Vascular invasion | 0.0006 | 0.272 | 1.256 | 0.836–1.886 | |
Neural invasion | 0.0388 | 0.240 | 1.306 | 0.837–2.037 | |
Pleural invasion | 0.0016 | 0.659 | 1.094 | 0.733–1.633 | |
Differentiation | |||||
Low | – | – | – | – | |
Moderate | 0.4616 | 0.289 | 0.834 | 0.595–1.167 | |
Well | 0.0319 | 0.021 | 0.436 | 0.215–0.882 | |
STAS | <0.001 | 0.026 | 1.470 | 1.046–2.066 | |
Stage | |||||
I | – | – | – | – | |
II | 0.0011 | 0.008 | 1.792 | 1.165–2.758 | |
III | <0.001 | <0.001 | 3.148 | 2.056–4.819 |
HR, hazard ratio; CI, confidence interval; STAS, spread through air spaces.
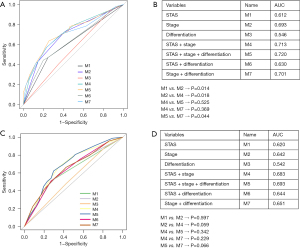
Establishment and clinical application of the nomogram
To provide a clinically useful tool for predicting prognosis, we constructed a nomogram integrating multiple clinicopathological risk factors associated with PFS, including STAS, AJCC staging, and tumor differentiation (Figure 3). Nomograms, also known as alignment diagrams, are a commonly used tool for estimating prognosis in oncology and medicine. Based on the results of the multivariate analysis, multiple predictive indexes are integrated, distributed according to a certain proportion, and the relationship between outcome predictions is visualized among variables in the form of graphics. At the same time, the length of the line can be used to express the impact of different variables on the outcome, as well as the impact of different values of variables on the outcome, so as to advance the pursuit of personalized medicine. The calibration curve showed that the nomogram performed well, and the predicted 3- or 5-year PFS from the nomogram was in good agreement with the Kaplan-Meier prediction (Figure 4). DCA was used to evaluate the clinical potential of the predictive models by quantifying the net benefit. We evaluated the clinical utility of the above seven models (Figure 2) using DCA. The threshold probability for a patient to choose a treatment suggests how the patient weighs the relative harms of false-positive and false-negative predictions. The net benefit was calculated by subtracting the proportion of all false-positive patients from the proportion of true-positive patients (20). Through DCA, it was found that the clinical application potential of AJCC staging combined with STAS was higher than that of AJCC staging alone. When tumor differentiation was included, the model showed higher potential for clinical application, as the threshold probability range for 3- or 5-year PFS compared to full- or no-treatment regimens ensured a better net gain (Figure 5).
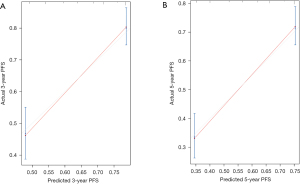
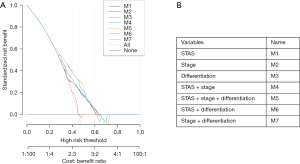
Discussion
The concept of STAS was clearly pointed out by the WHO in the pathological classification of lung cancer in 2015 as a new tumor invasion mode (4). Previously, Onozato et al. (21) had found this phenomenon in their study as an isolated, massive collection of tumor cells with vague micropapillary structures that exist in the alveolar air spaces, called tumor islands. Tumor islands are located around the lesion, separated from the main tumor by at least a few alveoli, and are seen in lung adenocarcinomas. Kadota et al. (18) later described the components of STAS in detail: “Single cells are defined as single tumor cells without cohesion in the alveolar space; micropapillary clusters are defined as the absence of a central fibrovascular core in the papillary structure; solid cell nests are defined as collections of tumor cells filling the alveolar spaces”. Morphologically, these tumor cells are located in the alveolar space, such as micropapillary clusters, solid cell nests, or individual tumor cells, as opposed to adherent growth in which tumor cells grow linearly along the alveolar wall surface. The degree of packing varies from massive cellular infiltration to inconspicuous single cells or micropapillary clusters that are sometimes difficult to distinguish from alveolar macrophages. In lung squamous cell carcinoma, Lu et al. (22) found that all STAS lesions consist of solid cell nests. STAS was initially identified in lung adenocarcinoma, but with subsequent extensive studies, STAS has also been found in other histological types of lung cancer, including lung squamous cell carcinoma, pleomorphic carcinoma, invasive mucinous adenocarcinoma, neuroendocrine tumor, and lymphoepithelioma-like carcinoma, among others. Chae et al. (23) found that the positive rate of STAS in neuroendocrine tumors (54/77, of which STAS was most common in small cell carcinoma) was higher than that of other previously reported tumors. At present, most studies focus on lung adenocarcinoma with a higher incidence, and studies related to STAS have reported that STAS is closely related to poor prognosis. As for research on the relationship between lung squamous cell carcinoma and STAS, only a small number of English studies have directly investigated STAS and lung squamous cell carcinoma (22,24,25) since 2015. According to the 2020 Global Cancer Center Annual work report (26), the 5-year relative survival rate of primary lung cancer was extremely low, only about 19.7%, which was about 3% higher than 10 years ago. The correlation between STAS and prognosis has been mentioned in many meta-analyses. An analysis of 8 studies before April 2018 included by Wang et al. (27) showed that STAS was associated with poor RFS and OS. An analysis of 14 studies before August 2018 included by Chen et al. (28) showed that STAS was also associated with poor RFS and OS in NSCLC. The analysis of 12 studies from 2015 to 2018 included by Liu et al. (29) showed that STAS was related to the decline of 5-year RFS. Yang et al. (30) included 11 studies involving patients with stage I lung adenocarcinoma before December 2020, and the analysis showed that STAS was an independent prognostic risk factor, and its high expression could lead to a high 5-year recurrence rate. Li et al. (31) included 13 studies of stage I NSCLC involving surgical procedures before March 2021, and the analysis showed that there was a significant correlation between STAS and RFS. In order to better assist clinical decision-making and individualized medical treatment, we analyzed a group of postoperative patients with lung squamous cell carcinoma to study STAS, and developed a nomogram that integrates STAS, AJCC staging, and other clinicopathological risk factors related to PFS to evaluate 3- and 5-year PFS in postoperative patients with lung squamous cell carcinoma. In this study, we divided the discovery and validation cohorts into STAS-negative/positive groups based on the description of STAS mentioned above. In the discovery cohort, patients in the STAS-negative group had significantly higher 5-year PFS than the STAS-positive group. Similar results were also observed in the validation cohort. Furthermore, we validated the potential value of STAS in predicting patient outcomes in the validation cohort. Multivariate analysis showed that STAS was an independent prognostic factor for PFS after adjustment for clinicopathological variables. This is consistent with the findings of Lu et al. (22) that STAS is an independent prognostic factor for recurrence and survival in postoperative patients with lung squamous cell carcinoma in North America, and that STAS is associated with poor prognosis in Japanese patients with postoperative lung squamous cell carcinoma as shown by Kadota et al. (25). Yanagawa et al. (24) further studied the correlation between STAS and lung cancer stage. Multivariate analysis showed that the presence of STAS was an independent predictor of recurrence in stage I lung squamous cell carcinoma, but not in stage II and stage III. In this study, the prediction of PFS by combining AJCC staging and STAS was better than using the AJCC staging system alone. The calibration curve showed that there was a good correlation between the predicted survival time and the actual survival time. DCA showed that the nomogram had high potential for clinical application.
A previous study has proposed that “STAS is an artificial product produced by knife face diffusion” (32). Part of the reason for the artifact comes from the unique anatomical structure of lung specimens, including the collapse of lung tissue caused by surgery and the contraction of smooth muscle ex vivo, which is inevitable, though other reasons are worthy of further attention. In the process of tumor section processing, tissue fragments and individual cells may spread with the cutting of the knife face. The concept of spreading through a knife surface (STAKS) was first proposed by Thunnissen et al. (32). They believe that artifacts from knife cutting are real, and that each continuous cut increases the mass of free-floating tumors. STAKS’s explanation for the association between STAS and histological types with poor prognosis is that tumor cells tend to separate. They suggest that about half of the reported lung adenocarcinomas have a frequency of STAS exactly in line with the possible frequency of STAKS. Blaauwgeers et al. (33) proposed that a typical cutter or scalpel blade is 30 times wider than a human cell, which may cause the cell to move along the knife path during the cutting process. Through a prospective study, they proved that the existence of free cells in lung cancer specimens is an induced and reproducible phenomenon, that is, the floating tumor cell mass is entirely caused by mechanical force. The spread of benign and malignant cells in the lung is likely to be caused by the mechanical force exerted on the tissue during surgery and/or dissection of the specimen. Since the lung tissue is mainly composed of air, it is highly likely that displaced cells will exist in the air cavity. This may also explain the relatively low frequency of intravascular and endobronchial fragments compared with intra-alveolar fragments. However, Lu et al. (22) proposed that in STAS, tumor cell nests have a smooth boundary and can be seen in a continuous pattern from the edge of the tumor to the farthest location. Artifacts are more likely to show jagged edges and random distribution on sections, such as isolated tumor fragments on tissue sections. In addition, in specimens of lung adenocarcinoma with STAS as the main histological pattern, it was observed that the area of floating tumor mass of STAS was not the area while the knife cutting through the main tumor. After Gross et al. (34) found STAS in the first surgical resection (R0 resection), several tumor cell clusters could still be found in the air cavity of the normal lung tissue resected in the subsequent second surgery. Metovic et al. (35) confirmed through a prospective study that there was no significant difference in the incidence of STAS between fresh tissues and formalin-fixed tissues, and there was no obvious dominant distribution of STAS in the air cavity of the lung parenchyma before and after the knife cut through the tumor. The results of these two studies strongly refute that STAS is a man-made event related to the operating procedure of pathologists.
In their review, Ikeda et al. (36) proposed other issues related to STAS recognition, such as the possibility of STAS recognition on frozen sections, and morphological and molecular properties of STAS. For preoperative prediction, ground glass opacity (37), solid component (38,39), and metabolic tumor volume (MTV)/computed tomography volume (CTV) >1 on 18F-fluorodeoxyglucose (FDG) positron emission tomography/computed tomography (PET/CT) (40) is associated with STAS. The prediction models (41-44) established by radiomics signatures can effectively predict STAS. With regard to intraoperative prediction, the diagnostic performance of frozen sections (45,46) is unfavorable due to their low sensitivity. With respect to morphological properties, micropapillary-predominant pattern (38,47-49) and solid-predominant pattern (47,48) are common in STAS. Nakajima et al. (50) found that cribriform pattern was also related to STAS. At the genetic level (47), epidermal growth factor receptor (EGFR), tumor protein p53 (TP53), Kirsten rat sarcoma viral oncogene (KRAS), anaplastic lymphoma kinase (ALK), and ROS proto-oncogene 1 (ROS1) are the 5 most common changes in STAS-positive lung adenocarcinoma. Other related studies on CD68+ tumor-associated macrophages (TAMs) (51) and epithelial-mesenchymal transition (EMT) (52) have also revealed their close relationship with STAS.
Although the nomogram combined with STAS had certain accuracy in predicting the survival of postoperative patients with lung squamous cell carcinoma, our current study still has some limitations. Firstly, this was a retrospective study, which may have a selection bias in population selection, and further prospective studies are needed to verify the findings. Secondly, the nomogram was verified internally, and subsequent verification should be performed in an external independent cohort. Furthermore, pathologists doubt whether the detection rate of STAS is affected by extensive sampling of peritumoral lung parenchyma. Since there is no consensus (8,23,53,54) on the definition of the degree of STAS, we did not conduct a graded study of STAS.
Conclusions
In summary, we developed and verified a nomogram that combines STAS and clinicopathological features, which can accurately predict the prognosis of postoperative patients with lung squamous cell carcinoma to a certain extent. Using an accurate prognostic model to predict patients’ survival will be of great help in choosing the best treatment strategy and individualizing patient treatment.
Acknowledgments
Funding: This study was supported by a grant from Major Science and Technology Innovation in 2025 Projects of Ningbo, China (No. 2019B10039) and Ningbo Clinical Research Center for Thoracic & Breast Neoplasms (No. 2021L002).
Footnote
Reporting Checklist: The authors have completed the TRIPOD reporting checklist. Available at https://jtd.amegroups.com/article/view/10.21037/jtd-22-1065/rc
Data Sharing Statement: Available at https://jtd.amegroups.com/article/view/10.21037/jtd-22-1065/dss
Conflicts of Interest: All authors have completed the ICMJE uniform disclosure form (available at https://jtd.amegroups.com/article/view/10.21037/jtd-22-1065/coif). The authors have no conflicts of interest to declare.
Ethical Statement: The authors are accountable for all aspects of the work in ensuring that questions related to the accuracy or integrity of any part of the work are appropriately investigated and resolved. The study was conducted in accordance with the Declaration of Helsinki (as revised in 2013). The study was approved by the ethics committee of Ningbo Medical Center Lihuili Hospital (The Affiliated Lihuili Hospital, Ningbo University) (No. KY2019PJ058) and individual consent for this retrospective analysis was waived.
Open Access Statement: This is an Open Access article distributed in accordance with the Creative Commons Attribution-NonCommercial-NoDerivs 4.0 International License (CC BY-NC-ND 4.0), which permits the non-commercial replication and distribution of the article with the strict proviso that no changes or edits are made and the original work is properly cited (including links to both the formal publication through the relevant DOI and the license). See: https://creativecommons.org/licenses/by-nc-nd/4.0/.
References
- Sung H, Ferlay J, Siegel RL, et al. Global Cancer Statistics 2020: GLOBOCAN Estimates of Incidence and Mortality Worldwide for 36 Cancers in 185 Countries. CA Cancer J Clin 2021;71:209-49. [Crossref] [PubMed]
- Schegoleva AA, Khozyainova AA, Fedorov AA, et al. Prognosis of Different Types of Non-Small Cell Lung Cancer Progression: Current State and Perspectives. Cell Physiol Biochem 2021;55:29-48. [Crossref] [PubMed]
- Chassagnon G, Bennani S, Revel MP. New TNM classification of non-small cell lung cancer. Rev Pneumol Clin 2017;73:34-9. [Crossref] [PubMed]
- Travis WD, Brambilla E, Nicholson AG, et al. The 2015 World Health Organization Classification of Lung Tumors: Impact of Genetic, Clinical and Radiologic Advances Since the 2004 Classification. J Thorac Oncol 2015;10:1243-60. [Crossref] [PubMed]
- Nicholson AG, Tsao MS, Beasley MB, et al. The 2021 WHO Classification of Lung Tumors: Impact of Advances Since 2015. J Thorac Oncol 2022;17:362-87. [Crossref] [PubMed]
- Jung W, Chung JH, Yum S, et al. The differential prognostic impact of spread through air spaces in early-stage lung adenocarcinoma after lobectomy according to the pT descriptor. J Thorac Cardiovasc Surg 2022;163:277-84.e1. [Crossref] [PubMed]
- Xie H, Su H, Zhu E, et al. Morphological Subtypes of Tumor Spread Through Air Spaces in Non-Small Cell Lung Cancer: Prognostic Heterogeneity and Its Underlying Mechanism. Front Oncol 2021;11:608353. [Crossref] [PubMed]
- Han YB, Kim H, Mino-Kenudson M, et al. Tumor spread through air spaces (STAS): prognostic significance of grading in non-small cell lung cancer. Mod Pathol 2021;34:549-61. [Crossref] [PubMed]
- Chae M, Jeon JH, Chung JH, et al. Prognostic significance of tumor spread through air spaces in patients with stage IA part-solid lung adenocarcinoma after sublobar resection. Lung Cancer 2021;152:21-6. [Crossref] [PubMed]
- Lee MA, Kang J, Lee HY, et al. Spread through air spaces (STAS) in invasive mucinous adenocarcinoma of the lung: Incidence, prognostic impact, and prediction based on clinicoradiologic factors. Thorac Cancer 2020;11:3145-54. [Crossref] [PubMed]
- Ikeda T, Kadota K, Go T, et al. Segmentectomy Provides Comparable Outcomes to Lobectomy for Stage IA Non-small Cell Lung Cancer with Spread through Air Spaces. Semin Thorac Cardiovasc Surg 2022; Epub ahead of print. [Crossref] [PubMed]
- Chen S, Ye T, Yang S, et al. Prognostic implication of tumor spread through air spaces in patients with pathologic N0 lung adenocarcinoma. Lung Cancer 2022;164:33-8. [Crossref] [PubMed]
- Kagimoto A, Tsutani Y, Kushitani K, et al. Segmentectomy vs Lobectomy for Clinical Stage IA Lung Adenocarcinoma With Spread Through Air Spaces. Ann Thorac Surg 2021;112:935-43. [Crossref] [PubMed]
- Zombori T, Sejben A, Tiszlavicz L, et al. Architectural Grade Combined With Spread Through Air Spaces (STAS) Predicts Recurrence and is Suitable for Stratifying Patients Who Might Be Eligible for Lung Sparing Surgery for Stage I Adenocarcinomas. Pathol Oncol Res 2020;26:2451-8. [Crossref] [PubMed]
- Shiono S, Endo M, Suzuki K, et al. Spread through air spaces affects survival and recurrence of patients with clinical stage IA non-small cell lung cancer after wedge resection. J Thorac Dis 2020;12:2247-60. [Crossref] [PubMed]
- Chen D, Wang X, Zhang F, et al. Could tumor spread through air spaces benefit from adjuvant chemotherapy in stage I lung adenocarcinoma? A multi-institutional study. Ther Adv Med Oncol 2020;12:1758835920978147. [Crossref] [PubMed]
- Liu A, Hou F, Qin Y, et al. Predictive value of a prognostic model based on pathologic features in lung invasive adenocarcinoma. Lung cancer 2019;131:14-22. [Crossref] [PubMed]
- Kadota K, Nitadori JI, Sima CS, et al. Tumor Spread through Air Spaces is an Important Pattern of Invasion and Impacts the Frequency and Location of Recurrences after Limited Resection for Small Stage I Lung Adenocarcinomas. J Thorac Oncol 2015;10:806-14. [Crossref] [PubMed]
- Kadota K, Kushida Y, Kagawa S, et al. Limited Resection Is Associated With a Higher Risk of Locoregional Recurrence than Lobectomy in Stage I Lung Adenocarcinoma With Tumor Spread Through Air Spaces. Am J Surg Pathol 2019;43:1033-41. [Crossref] [PubMed]
- Meng J, Zhang J, Xiu Y, et al. Prognostic value of an immunohistochemical signature in patients with esophageal squamous cell carcinoma undergoing radical esophagectomy. Mol Oncol 2018;12:196-207. [Crossref] [PubMed]
- Onozato ML, Kovach AE, Yeap BY, et al. Tumor islands in resected early-stage lung adenocarcinomas are associated with unique clinicopathologic and molecular characteristics and worse prognosis. Am J Surg Pathol 2013;37:287-94. [Crossref] [PubMed]
- Lu S, Tan KS, Kadota K, et al. Spread through Air Spaces (STAS) Is an Independent Predictor of Recurrence and Lung Cancer-Specific Death in Squamous Cell Carcinoma. J Thorac Oncol 2017;12:223-34. [Crossref] [PubMed]
- Chae M, Cho S, Chung JH, et al. Poor Prognosis of Grade 2 Spread Through Air Spaces in Neuroendocrine Tumors. J Chest Surg 2022;55:101-7. [Crossref] [PubMed]
- Yanagawa N, Shiono S, Endo M, et al. Tumor spread through air spaces is a useful predictor of recurrence and prognosis in stage I lung squamous cell carcinoma, but not in stage II and III. Lung Cancer 2018;120:14-21. [Crossref] [PubMed]
- Kadota K, Kushida Y, Katsuki N, et al. Tumor Spread Through Air Spaces Is an Independent Predictor of Recurrence-free Survival in Patients With Resected Lung Squamous Cell Carcinoma. Am J Surg Pathol 2017;41:1077-86. [Crossref] [PubMed]
- Pan F. Integrated prevention and treatment is an important strategy to improve the 5-year survival rate of cancer patients -- Tsinghua Tobacco Control and Lung Cancer Prevention and Control Forum. China Modern Medicine 2021;28:1-3.
- Wang S, Hao J, Qian C, et al. Tumor Spread Through Air Spaces Is a Survival Predictor in Non-Small-Cell Lung Cancer. Clin Lung Cancer 2019;20:e584-91. [Crossref] [PubMed]
- Chen D, Mao Y, Wen J, et al. Tumor Spread Through Air Spaces in Non-Small Cell Lung Cancer: A Systematic Review and Meta-Analysis. Ann Thorac Surg 2019;108:945-54. [Crossref] [PubMed]
- Liu H, Yin Q, Yang G, et al. Prognostic Impact of Tumor Spread Through Air Spaces in Non-small Cell Lung Cancers: a Meta-Analysis Including 3564 Patients. Pathol Oncol Res 2019;25:1303-10. [Crossref] [PubMed]
- Yang Y, Xie X, Wang Y, et al. A systematic review and meta-analysis of the influence of STAS on the long-term prognosis of stage I lung adenocarcinoma. Transl Cancer Res 2021;10:2428-36. [Crossref] [PubMed]
- Li J, Wang Y, Li J, et al. Meta-analysis of Lobectomy and Sublobar Resection for Stage I Non-small Cell Lung Cancer With Spread Through Air Spaces. Clin Lung Cancer 2022;23:208-13. [Crossref] [PubMed]
- Thunnissen E, Blaauwgeers HJ, de Cuba EM, et al. Ex Vivo Artifacts and Histopathologic Pitfalls in the Lung. Arch Pathol Lab Med 2016;140:212-20. [Crossref] [PubMed]
- Blaauwgeers H, Flieder D, Warth A, et al. A Prospective Study of Loose Tissue Fragments in Non-Small Cell Lung Cancer Resection Specimens: An Alternative View to "Spread Through Air Spaces". Am J Surg Pathol 2017;41:1226-30. [Crossref] [PubMed]
- Gross DJ, Hsieh MS, Li Y, et al. Spread Through Air Spaces (STAS) in Non-Small Cell Lung Carcinoma: Evidence Supportive of an In Vivo Phenomenon. Am J Surg Pathol 2021;45:1509-15. [Crossref] [PubMed]
- Metovic J, Falco EC, Vissio E, et al. Gross Specimen Handling Procedures Do Not Impact the Occurrence of Spread Through Air Spaces (STAS) in Lung Cancer. Am J Surg Pathol 2021;45:215-22. [Crossref] [PubMed]
- Ikeda T, Kadota K, Go T, et al. Current status and perspectives of spread through air spaces in lung cancer. Thorac Cancer 2021;12:1639-46. [Crossref] [PubMed]
- Zhong Y, Xu Y, Deng J, et al. Prognostic impact of tumour spread through air space in radiological subsolid and pure solid lung adenocarcinoma. Eur J Cardiothorac Surg 2021;59:624-32. [Crossref] [PubMed]
- Yi E, Lee JH, Jung Y, et al. Clinical implication of tumour spread through air spaces in pathological stage I lung adenocarcinoma treated with lobectomy. Interact Cardiovasc Thorac Surg 2021;32:64-72. [Crossref] [PubMed]
- Qi L, Xue K, Cai Y, et al. Predictors of CT Morphologic Features to Identify Spread Through Air Spaces Preoperatively in Small-Sized Lung Adenocarcinoma. Front Oncol 2020;10:548430. [Crossref] [PubMed]
- Falay O, Selçukbiricik F, Tanju S, et al. The prediction of spread through air spaces with preoperative 18F-FDG PET/CT in cases with primary lung adenocarcinoma, its effect on the decision for an adjuvant treatment and its prognostic role. Nucl Med Commun 2021;42:922-7. [Crossref] [PubMed]
- Liao G, Huang L, Wu S, et al. Preoperative CT-based peritumoral and tumoral radiomic features prediction for tumor spread through air spaces in clinical stage I lung adenocarcinoma. Lung Cancer 2022;163:87-95. [Crossref] [PubMed]
- Qi L, Li X, He L, et al. Comparison of Diagnostic Performance of Spread Through Airspaces of Lung Adenocarcinoma Based on Morphological Analysis and Perinodular and Intranodular Radiomic Features on Chest CT Images. Front Oncol 2021;11:654413. [Crossref] [PubMed]
- Onozato Y, Nakajima T, Yokota H, et al. Radiomics is feasible for prediction of spread through air spaces in patients with nonsmall cell lung cancer. Sci Rep 2021;11:13526. [Crossref] [PubMed]
- Chen LW, Lin MW, Hsieh MS, et al. Radiomic Values from High-Grade Subtypes to Predict Spread Through Air Spaces in Lung Adenocarcinoma. Ann Thorac Surg 2022;114:999-1006. [Crossref] [PubMed]
- Zhou F, Villalba JA, Sayo TMS, et al. Assessment of the feasibility of frozen sections for the detection of spread through air spaces (STAS) in pulmonary adenocarcinoma. Mod Pathol 2022;35:210-7. [Crossref] [PubMed]
- Villalba JA, Shih AR, Sayo TMS, et al. Accuracy and Reproducibility of Intraoperative Assessment on Tumor Spread Through Air Spaces in Stage 1 Lung Adenocarcinomas. J Thorac Oncol 2021;16:619-29. [Crossref] [PubMed]
- Tian Y, Feng J, Jiang L, et al. Integration of clinicopathological and mutational data offers insight into lung cancer with tumor spread through air spaces. Ann Transl Med 2021;9:985. [Crossref] [PubMed]
- Cao L, Jia M, Sun PL, et al. Histopathologic features from preoperative biopsies to predict spread through air spaces in early-stage lung adenocarcinoma: a retrospective study. BMC Cancer 2021;21:913. [Crossref] [PubMed]
- Alvarez Moreno JC, Aljamal AA, Bahmad HF, et al. Correlation between spread through air spaces (STAS) and other clinicopathological parameters in lung cancer. Pathol Res Pract 2021;220:153376. [Crossref] [PubMed]
- Nakajima N, Yoshizawa A, Rokutan-Kurata M, et al. Prognostic significance of cribriform adenocarcinoma of the lung: validation analysis of 1,057 Japanese patients with resected lung adenocarcinoma and a review of the literature. Transl Lung Cancer Res 2021;10:117-27. [Crossref] [PubMed]
- Yoshida C, Kadota K, Ikeda T, et al. Tumor-associated macrophage infiltration is associated with a higher rate of tumor spread through air spaces in resected lung adenocarcinomas. Lung Cancer 2021;158:91-6. [Crossref] [PubMed]
- Ikeda T, Kadota K, Yoshida C, et al. The epithelial-mesenchymal transition phenotype is associated with the frequency of tumor spread through air spaces (STAS) and a High risk of recurrence after resection of lung carcinoma. Lung Cancer 2021;153:49-55. [Crossref] [PubMed]
- Uruga H, Fujii T, Fujimori S, et al. Semiquantitative Assessment of Tumor Spread through Air Spaces (STAS) in Early-Stage Lung Adenocarcinomas. J Thorac Oncol 2017;12:1046-51. [Crossref] [PubMed]
- Warth A, Muley T, Kossakowski CA, et al. Prognostic Impact of Intra-alveolar Tumor Spread in Pulmonary Adenocarcinoma. Am J Surg Pathol 2015;39:793-801. [Crossref] [PubMed]
(English Language Editor: C. Betlazar-Maseh)