The association of coagulation indicators with in-hospital acute kidney injury and malignant events of patients with acute aortic dissection: a retrospective cohort study
Highlight box
Key findings
• The coagulation indicators had a correlation with in-hospital AKI and malignant events in patients with AAD.
• The worse the coagulation dysfunction is, the higher the possibility of AKI in patients with AAD.
What is known and what is new?
• Coagulation dysfunction serves as a valuable diagnostic and prognostic marker for AAD, but also plays a role in the pathological mechanisms underlying AKI.
• The presence of coagulation dysfunction is associated with an increased risk of AKI and malignant events and the severity of coagulation dysfunction is positively correlated with the incidence of in-hospital AKI in AAD patients.
What is the implication, and what should change now?
• For those patients with AAD and coagulation dysfunction, kidney protection and treatment measures should be taken to avoid AKI.
• Coagulation indicators can be used for the early identification of patients at high-risk for AKI.
Introduction
Acute aortic dissection (AAD) is one of the most devastating cardiovascular diseases and causes death in about 1–2% of patients in the first 24–48 hours after AAD (1,2). In previous reports, the prevalence of acute kidney injury (AKI) was noted to be 25–55% in AAD (3,4), with AKI complicating the course of 50% to 60% of those admitted to the intensive care unit (ICU); AKI was also reported to lead to poor prognosis, with increased short-term mortality and multi-organ failure (3,5,6).
Previous research has shown the potential utility of abnormal coagulation indicators as diagnostic and prognostic markers for AAD (7-11). The onset of AAD may result in endothelial injury due to the formation of the false lumen, which can activate the coagulation pathway and lead to coagulation dysfunction. When coagulation factors and fibrinolytic substances are continuously consumed, abnormal coagulation function inevitably occurs, putting the body in a state of hypocoagulability as reflected by coagulation indicators (12). More evidence is needed on the relationship between coagulopathy and in-hospital AKI and malignant events in AAD patients.
Furthermore, coagulation indicators have also been identified as potential mechanisms of AKI in other diseases, such as sepsis and poisoning (12,13). Coagulation dysfunction, together with inflammation, hypoperfusion injury and other mechanisms, may contribute to the development of AKI and increase the risk of mortality (5,14,15). While current research on the mechanisms of AKI and mortality in patients with AAD has primarily focused on renal injury, hypoperfusion, ischemia/reperfusion, inflammation, and the use of contrast agents in interventional therapy (5,13-16), the role of coagulation dysfunction has yet to be fully explored, which provides new insights into the relationship between coagulation dysfunction and in-hospital AKI and malignant events in patients with AAD.
Therefore, to determine whether coagulopathy contributes to in-hospital AKI and malignant events in patients with AAD, we aimed to determine whether it contributes to the development of AKI and malignant events after AAD by assessing the presence of coagulation dysfunction at admission. The results of this study provide valuable clinical insights for the diagnosis and treatment of patients with AAD by allowing for the timely detection and intervention of those at risk of in-hospital AKI and malignant events. We present the following article in accordance with the STARD reporting checklist (available at https://jtd.amegroups.com/article/view/10.21037/jtd-23-38/rc).
Methods
Study design and population
This was a single-center, observational study conducted from January 2015 to October 2020 that enrolled consecutive patients with AAD admitted to the First Affiliated Hospital of Shantou University Medical College. All patients with acute aortic syndromes (e.g., intramural hematoma, penetrating atherosclerotic ulcer) referred to the First Affiliated Hospital of Shantou University Medical College during the study period were registered. Adult patients with AAD symptom duration <48 hours as confirmed by computed tomography for first AAD diagnosis were included. Patients were excluded if they lacked a coagulation examination or had insufficient clinical record. The inclusion flowchart is presented in Figure 1.
Coagulation dysfunction was considered present when one or more of the coagulation indicators, including prothrombin time (PT), thrombin time (TT), international normalized ratio (INR), activated partial thromboplastin time (APTT), fibrinogen content (Fib), and platelet count (PLT) as shown in Table 1, were found to be abnormal (17). The patients with coagulation dysfunction were grouped as the abnormal coagulation group. Abnormal coagulation score (ACS) corresponded with the sum of abnormal coagulation indicator scores. D-dimer and fibrin degradation products were not included, as these have been confirmed to be part of the diagnostic basis for AAD (8,18).
Table 1
Indicator | Reference value | Diagnostic criteria |
---|---|---|
PT | 10.5–14 s | Extension >3 s |
TT | 14–21 s | Extension >3 s |
INR | 0.8–1.2 | >1.3 |
APTT | 23.3–32.5 s | Extension >10 s |
Fib | 2–4 g/L | <2 g/L |
PLT | [125–350]×109/L | <125×109/L |
PT, prothrombin time; TT, thrombin time; INR, international normalized ratio; APTT, activated partial thromboplastin time; Fib, fibrinogen content; PLT, platelet count.
Previous studies have not systematically investigated the incidence of AKI in patients with AAD in relation to coagulation dysfunction. Data collected were validated by patient acceptable symptom status (PASS) at a two-sided 5% significance level and adequate statistical power (1-β ≥0.90) was ensured.
Variables and end points
We collected patient data from electronic medical records and recorded them on a previously designed e-sheet with 4 parts: (I) demographic data, including age and sex; (II) admission status data, including diagnosis, comorbidities (hypertension, etc.), and clinical features, such as ischemia performance and number of involved renal arteries, which are known confounding factors in clinic (5,6); (III) admission laboratory data, including serum creatinine (SCr), APTT, PT, INR, TT, Fib, and PLT; and (IV) the primary outcome of AKI at admission. We defined patients with AKI according to the criteria of the Kidney Disease Improving Global Outcomes (KDIGO) classification criteria during admission (19). The lowest SCr recorded at the hospital 1 year earlier was used as baseline. If no baseline SCr was recorded, baseline SCr was estimated according to the KDIGO guideline, with an assumed baseline estimated glomerular filtration rate of 75 mL/min per 1.73 m2 (12). The secondary outcomes included in-hospital all-cause mortality, interlayer fracture, and cardiac arrest or the need for ventricular fibrillation for cardiopulmonary resuscitation, which were defined as malignant events.
Ethical considerations
The study was conducted in accordance with the Declaration of Helsinki (as revised in 2013). The study was approved by the Ethics Committee for Clinical Research at the First Affiliated Hospital of Shantou University Medical College (No. B-2023-003). Individual consent for this retrospective analysis was waived.
Statistical analysis
All the data were divided into two categories: continuous variables and categorical data. The measurement data are expressed as the mean ± SD or median [interquartile range (IQR)]. Categorical data are expressed as the number (percentage). Continuous variables were analyzed using Student t test, and categorical variables were analyzed using chi-squared or Fisher exact test. To estimate the coagulation dysfunction of AKI and malignant events, odds ratios (ORs) and 95% CIs were calculated with univariable analysis and multivariable adjustments in the following models to reduce the effect of known possible confounders: model 1—adjusted for age, gender, acute aortic dissention (Stanford classification), smoking history, drinking history, systolic blood pressure (SBP), diastolic blood pressure (DBP), and comorbidities (including hypertension, Marfan syndrome, heart surgery history, arteriosclerosis, coronary heart disease, aortic valve disease, and diabetes mellitus type 2); model 2—adjusted model 1 + murmur in the aortic valve auscultation area, organ or limb ischemia, hypotensive shock, number of renal arteries involved, aneurysm on imaging, ulcer of the aorta, and aortic intermural hematoma; and model 3—adjusted model 2 + white blood cell count.
In addition, propensity score matching (PSM), using nearest-neighbor matching (1:1) within a caliper width of 0.02 SD without replacement, was performed between the coagulation dysfunction groups based on the estimated propensity scores. Furthermore, to confirm the robustness of the results, PSM was performed on the same results as a sensitivity analysis.
To further clarify the contribution rate of each coagulation indicator, chi-squared or Fisher exact test was used to compare the dysfunction of each coagulation indicator across the different prognosis groups. Receiver operating characteristic (ROC) curve analysis was then used to evaluate the value of each coagulative indicator and ACS for predicting the in-hospital AKI and malignant events.
Statistical analyses were performed with SPSS version 27.0 and PASS version 15. A two-sided P value of <0.05 was considered to denote the presence of a statistically significant difference.
Results
Patients’ baseline characteristics
Table 2 shows the demographics, medical history, clinical features, and laboratory data of the participants. Ultimately, with a two-side 5% significance level, the sample size of 309 and 178 patients and the incidence of AKI was 0.71 and 0.84 in the normal and abnormal coagulation groups, respectively, the statistical power (1-β) was 0.93. Men were in the majority with a percentage of 80%. The Stanford classification was roughly symmetrical, and the proportion of Stanford A in the abnormal coagulation group was significantly higher than that the normal coagulation group. Before PSM, these two groups appeared similar in terms of age, gender ratio, and medical history. The percentage of patients with poor ischemia performance and involved renal was not different between the coagulation groups. However, a greater portion of patients in the abnormal coagulation group had AAD (Stanford A), hyperuricemia, a history of heart surgery history, and lower blood pressure. After PSM, baseline characteristics were balanced between the two groups (Table S1).
Table 2
Characteristics | Value (n=487) | Normal coagulation (n=309) | Abnormal coagulation (n=178) | P value |
---|---|---|---|---|
Age (years), median [IQR] | 57 [48–64] | 57 [48–64] | 57 [49–64] | 0.638 |
Male, n (%) | 394 (80.9) | 245 (79.3) | 149 (83.7) | 0.232 |
Stanford A, n (%) | 270 (55.4) | 147 (47.6) | 123 (69.1) | 0.000*** |
Previous history, n (%) | ||||
Smoking | 324 (66.5) | 204 (66.0) | 120 (67.4) | 0.753 |
Drinking | 78 (16.0) | 46 (14.9) | 32 (18.0) | 0.370 |
Hypertension | 431 (88.5) | 280 (90.6) | 151 (84.8) | 0.054 |
Marfan syndrome | 11 (2.3) | 7 (2.3) | 4 (2.2) | 0.990 |
Heart surgery history | 8 (1.6) | 1 (0.3) | 7 (3.9) | 0.003** |
Arteriosclerosis | 36 (7.4) | 25 (8.1) | 11 (6.2) | 0.438 |
Coronary heart disease | 36 (7.4) | 23 (7.4) | 13 (7.3) | 0.955 |
Cardiac insufficiency | 17 (3.5) | 11 (3.6) | 6 (3.4) | 0.913 |
Atrial fibrillation | 14 (2.9) | 6 (1.9) | 8 (4.5) | 0.104 |
Aortic valve disease | 27 (5.5) | 18 (5.8) | 9 (5.1) | 0.721 |
Diabetes mellitus type 2 | 42 (8.6) | 32 (10.4) | 10 (5.6) | 0.073 |
COPD/asthma | 11 (2.3) | 9 (2.9) | 2 (1.1) | 0.201 |
Cerebrovascular disease | 21 (4.3) | 13 (4.2) | 8 (4.5) | 0.881 |
Anemia | 167 (34.3) | 100 (32.4) | 67 (37.6) | 0.201 |
Hypoproteinemia | 412 (84.6) | 267 (86.4) | 145 (81.5) | 0.145 |
Hyperuricemia | 206 (42.3) | 113 (36.6) | 93 (52.2) | 0.000*** |
Clinical feature | ||||
Systolic blood pressure (mmHg), mean ± SD | 164±34.1 | 167.2±33.4 | 158.7±34.7 | 0.008** |
Diastolic blood pressure (mmHg), mean ± SD | 91.9±23.0 | 94.3±22.0 | 87.8±24.1 | 0.003** |
BP asymmetry in extremities, n (%) | 391 (80.3) | 247 (79.9) | 19.1 (80.9) | 0.797 |
Pulse press (beat/min), mean ± SD | 72.2±21.2 | 72.9±20.9 | 70.9±21.6 | 0.473 |
Heart rate (beat/min), mean ± SD | 78.8±16.9 | 79.5±15.5 | 77.7±19.0 | 0.087 |
Chest pain, n (%) | 398 (81.7) | 247 (79.9) | 151 (84.8) | 0.178 |
Back pain, n (%) | 355 (72.9) | 217 (70.2) | 138 (77.5) | 0.081 |
Abdominal pain, n (%) | 110 (22.6) | 77 (24.9) | 33 (18.5) | 0.105 |
Murmur in aortic valve auscultation area, n (%) | 100 (20.5) | 53 (17.2) | 47 (26.4) | 0.015* |
Organ or limb ischemia, n (%) | 162 (33.3) | 98 (31.7) | 64 (36.0) | 0.339 |
Hypotensive shock, n (%) | 20 (4.1) | 9 (2.9) | 11 (6.2) | 0.080 |
Laboratory findings | ||||
ABO blood type, n (%) | 0.161 | |||
A | 126 (26.6) | 84 (27.9) | 42 (24.3) | |
B | 124 (26.2) | 75 (24.9) | 49 (28.3) | |
AB | 30 (6.3) | 24 (8.0) | 6 (3.5) | |
O | 194 (40.9) | 118 (39.2) | 76 (43.9) | |
White blood cell (109/L), mean ± SD | 14.1±4.4 | 13.6±4.2 | 15.1±4.7 | 0.000*** |
Hemoglobin (g/L), median (IQR) | 133.0 (122.0–145.0) | 133.0 (121.0–144.0) | 133.0 (122.8–147.3) | 0.512 |
Number of renal arteries involved, n (%) | 0.396 | |||
1 | 244 (50.1) | 157 (50.8) | 87 (48.9) | |
2 | 41 (8.4) | 22 (7.1) | 19 (10.7) | |
Thrombosis, n (%) | 129 (27.6) | 176 (59.3) | 81 (47.4) | 0.013* |
Aneurysm on imaging, n (%) | 57 (12.2) | 37 (12.5) | 20 (11.7) | 0.808 |
Ulcer of aorta, n (%) | 46 (9.8) | 34 (11.4) | 12 (7.0) | 0.121 |
Aortic intermural hematoma, n (%) | 179 (38.2) | 119 (40.1) | 60 (35.1) | 0.286 |
Pericardial effusion, n (%) | 70 (15.0) | 38 (12.8) | 32 (18.7) | 0.084 |
Pleural effusion, n (%) | 111 (23.7) | 74 (24.9) | 37 (21.6) | 0.422 |
Renal cyst, n (%) | 165 (35.2) | 108 (36.2) | 57 (33.3) | 0.526 |
End point, n (%) | ||||
Acute kidney injury | 369 (75.8) | 219 (70.9) | 150 (84.3) | 0.000*** |
Malignant events | 60 (12.3) | 28 (9.1) | 32 (18.0) | 0.004** |
In-hospital all-cause mortality | 51 (10.5) | 23 (7.4) | 28 (15.7) | 0.040* |
Interlayer fracture | 42 (8.6) | 19 (6.1) | 23 (12.9) | 0.010* |
Cardiac arrest/ventricular fibrillation | 38 (7.8) | 16 (5.2) | 22 (12.4) | 0.040* |
*, P<0.05; **, P<0.01; ***, P<0.001. IQR, interquartile range; SD, standard deviation; COPD, chronic obstructive pulmonary disease; BP, blood pressure.
Clinical outcomes
Univariate analysis (unadjusted) was carried out for all variables, from which we selected variables with statistical difference (P<0.05) for multivariate logistic regression analysis. The independent influence factors on in-hospital AKI included age, male sex, organ or limb ischemia, and ACS. AAD (Stanford A), hypotensive shock, blood type, and abnormal PT tended to influence the incidence of malignant events (Figure 2).
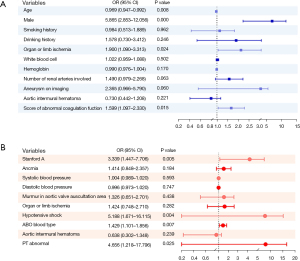
The incidence of AKI in the abnormal coagulation group was significantly higher than that in the normal coagulation group. ORs were calculated for AKI after adjustments were made for possible confounders in 3 models (model 1: OR 2.061, 95% CI: 1.214–3.501, P=0.007; model 2: OR 1.833, 95% CI: 1.058–3.177, P=0.031; model 3: OR 1.836, 95% CI: 1.048–3.216, P=0.034; PSM: OR 4.419, 95% CI: 2.650–7.370, P<0.001) (see in Table 3).
Table 3
AKI group | Malignant events group | ||||
---|---|---|---|---|---|
OR (95% CI) | P value | OR (95% CI) | P value | ||
Univariable | 2.202 (1.373–3.530) | 0.001** | 6.379 (1.884–21.598) | 0.003** | |
Multivariable | |||||
Model 1 | 2.061 (1.214–3.501) | 0.007** | 4.283 (0.983–18.665) | 0.053 | |
Model 2 | 1.833 (1.058–3.177) | 0.031* | 7.342 (1.467–36.749) | 0.015* | |
Model 3 | 1.836 (1.048–3.216) | 0.034* | 6.996 (1.377–35.537) | 0.019* | |
PSM | 4.419 (2.650–7.370) | <0.001*** | 8.969 (2.312–34.796) | 0.002** |
*, P<0.05; **, P<0.01; ***, P<0.001. AKI, acute kidney injury; AAD, acute aortic dissection; PT, prothrombin time; PSM, propensity score matching; OR, odds ratio; CI, confidence interval.
After adjustments were made for the covariates, the incidence of malignant events was found to be higher in the abnormal PT group (model 1: OR 4.283, 95% CI: 0.983–18.665, P=0.053; model 2: OR 7.342, 95% CI: 1.467–36.749, P=0.015; model 3: OR, 6.996, 95% CI: 1.377–35.537, P=0.019; PSM: OR 8.969, 95% CI: 2.312–34.796, P=0.002).
To compare the dysfunction of each coagulation indicator among the AKI groups and malignant events groups, Chi-squared and Fisher exact tests were used. These revealed PT, Fib, PLT, and abnormal coagulation to be statistically significant. In general, the higher the proportion of abnormal indicators was, the greater the possibility was of poor prognosis (Figure 3). To measure the contribution rate of each indicator, ROC analysis was conducted, which indicated that Fib was a useful prognostic method (AUC =0.649; P<0.001) for the coincidence of AKI but that ACS was even more valuable (AUC =0.688; P<0.001). There were no significant results in malignant event groups after ROC curve analysis was performed (Table 4).
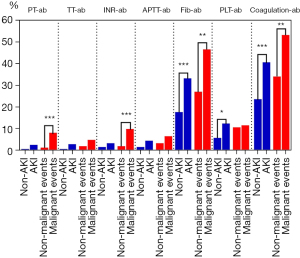
Table 4
Indicators | AUC | P value |
---|---|---|
PT abnormal | 0.519 | 0.570 |
TT abnormal | 0.519 | 0.580 |
INR abnormal | 0.521 | 0.537 |
APTT abnormal | 0.523 | 0.506 |
Fib abnormal | 0.649 | <0.001*** |
PLT abnormal | 0.563 | 0.063 |
Abnormal coagulation score | 0.688 | <0.001*** |
***, P<0.001. AKI, acute kidney injury; PT, prothrombin time; TT, thrombin time; INR, international normalized ratio; APTT, activated partial thromboplastin time; Fib, fibrinogen content; PLT, platelet count; AUC, area under the receiver operating characteristic curve.
Discussion
In this cohort study of patients with AAD undergoing AKI and malignant events, we demonstrated that abnormal coagulation was significantly associated with a poor prognosis. The results were consistent after multivariable adjustments in 3 models that considered comorbidities, hypoperfusion, inflammation, and ischemia/reperfusion, among other factors. After PSM, we found that patients with abnormal coagulation had about a 4.4 times greater possibility to develop AKI while patients with abnormal PT had an almost 9.0 times greater possibility to develop malignant events compared to their normal counterparts.
Coagulation dysfunction is not only a characteristic of AAD (5,7-11), but is the physiopathologic mechanism of AKI (6,13,14,16,20). Theoretically, when AAD occurs, endothelial injury may be accompanied by the formation of a false lumen, which may activate the coagulation pathway, precipitate a cascade reaction, and then activate the fibrinolytic system (12). When coagulation factors and fibrinolytic substances are consumed continuously, abnormal coagulation function will inevitably occur, placing the body in a state of hypocoagulability (21). It has also been found that aneurysms that consume platelets and generate coagulation fibers, PT, APTT, and TT may be prolonged, while fibrin degradation products may be reduced (21). This is consistent with the decline in Fib and PLT found in our study, which is suggestive of hyperfibrinolysis (12).
In addition, coagulation dysfunction may further produce microthrombi, which may produce thrombus deposits as they pass through renal vessels (14) and may further contribute to the AKI. Moreover, thrombin can participate in the activation of endothelial cells after injury. In an experiment using an animal model of AKI, it was confirmed that coagulation dysfunction could limit medullar capillary perfusion, leading to prolonged regional ischemia/hypoxia and hindering the repair and regeneration of tubular cells. In other studies, on thrombin targeted therapy, the improvement of renal microvascular circulation through the antithrombin effect could reflect the influence of abnormal coagulation on AKI (22). Thrombin production is higher in patients with worsening renal function, which is an independent risk factor for AKI in patients with anemia and may lead to microcirculation disturbance and tubule cell damage (23).
Other indicators in our study, including the prolonged APTT and TT, showed no significant difference, which is inconsistent with other research (7,9). This may be explained by the characteristics of coagulation. Coagulation is a cascade reaction, and various indicators influence and interact with each other. Considering a single factor in isolation makes it difficult to analyze its most salient characteristics. Moreover, further ROC analysis showed ACS to have the best performance in predicting the in-hospital AKI.
This paper identified a simple but useful system for predicting AKI by clinical coagulation dysfunction in patients with AAD. Above all, this will provide clinical ideas regarding the diagnosis and treatment of patients with AAD. For patients with coagulation dysfunction, kidney protection and treatment measures should be taken as soon as possible to avoid the occurrence of AKI. This system can be used for the early identification of high-risk patients, the early diagnosis of AKI, the stabilization of the hemodynamic parameters, the avoidance of nephrotoxic drugs, and the optimization of the use of contrast agents (6) which may be useful in preventing renal failure, reducing the length of hospital stay, and saving medical resources. Nevertheless, the mechanism of AKI is still controversial (5,13,16), while the mechanism underlying the association between coagulopathy and AKI is still unclear and should be investigated further.
Limitations
We used a single-center, retrospective design with a small sample size. It is necessary to conduct a prospective, multicenter trial to evaluate the relationship between coagulation indicators and AKI. Some literature shows that the KDIGO criteria are the most widely used for the definition of AKI, but the incidence of AKI calculated by the KDIGO appears to be too high.
Conclusions
Our study confirmed that the presence of coagulation dysfunction is associated with an increased risk of AKI and malignant events. It suggested that the severity of coagulation dysfunction is positively correlated with the incidence of in-hospital AKI in AAD patients. These results highlight the importance of considering coagulation dysfunction as a potential mechanism underlying the poor prognosis of AKI and malignant events in patients with AAD. Further research is needed to better understand the underlying mechanisms of coagulation dysfunction in this context, and to develop effective interventions for mitigating the risk of these adverse outcomes in AAD patients.
Acknowledgments
The authors wish to thank the enrolled patients for their participation and the research staff for their efforts in the study.
Funding: The study was partially supported by the 2020 Li Ka Shing Foundation Cross-Disciplinary Research Grant (No. L1111 2004).
Footnote
Reporting Checklist: The authors have completed the STARD reporting checklist. Available at https://jtd.amegroups.com/article/view/10.21037/jtd-23-38/rc
Data Sharing Statement: Available at https://jtd.amegroups.com/article/view/10.21037/jtd-23-38/dss
Conflicts of Interest: All authors have completed the ICMJE uniform disclosure form (available at https://jtd.amegroups.com/article/view/10.21037/jtd-23-38/coif). BW reports that the study was partially supported by the 2020 Li Ka Shing Foundation Cross-Disciplinary Research Grant (No. L1111 2004). The other authors have no conflicts of interest to declare.
Ethical Statement: The authors are accountable for all aspects of the work in ensuring that questions related to the accuracy or integrity of any part of the work are appropriately investigated and resolved. The study was conducted in accordance with the Declaration of Helsinki (as revised in 2013). The study was approved by the Ethics Committee for Clinical Research at the First Affiliated Hospital of Shantou University Medical College (No. B-2023-003). Individual consent for this retrospective analysis was waived.
Open Access Statement: This is an Open Access article distributed in accordance with the Creative Commons Attribution-NonCommercial-NoDerivs 4.0 International License (CC BY-NC-ND 4.0), which permits the non-commercial replication and distribution of the article with the strict proviso that no changes or edits are made and the original work is properly cited (including links to both the formal publication through the relevant DOI and the license). See: https://creativecommons.org/licenses/by-nc-nd/4.0/.
References
- Nienaber CA, Clough RE, Sakalihasan N, et al. Aortic dissection. Nat Rev Dis Primers 2016;2:16071. [Crossref] [PubMed]
- Zhu Y, Lingala B, Baiocchi M, et al. Type A Aortic Dissection-Experience Over 5 Decades: JACC Historical Breakthroughs in Perspective. J Am Coll Cardiol 2020;76:1703-13. [Crossref] [PubMed]
- Nadim MK, Forni LG, Bihorac A, et al. Cardiac and Vascular Surgery-Associated Acute Kidney Injury: The 20th International Consensus Conference of the ADQI (Acute Disease Quality Initiative) Group. J Am Heart Assoc 2018;7:e008834. [Crossref] [PubMed]
- Hoste EA, Bagshaw SM, Bellomo R, et al. Epidemiology of acute kidney injury in critically ill patients: the multinational AKI-EPI study. Intensive Care Med 2015;41:1411-23. [Crossref] [PubMed]
- Takahashi T, Hasegawa T, Hirata N, et al. Impact of acute kidney injury on in-hospital outcomes in patients with DeBakey type III acute aortic dissection. Am J Cardiol 2014;113:1904-10. [Crossref] [PubMed]
- Chen X, Bai M, Sun S, et al. Outcomes and risk management in type B aortic dissection patients with acute kidney injury: a concise review. Ren Fail 2021;43:585-96. [Crossref] [PubMed]
- Arima D, Suematsu Y, Yamada R, et al. Relationship of acute type A aortic dissection and disseminated intravascular coagulation. J Vasc Surg 2022;75:1553-1560.e1. [Crossref] [PubMed]
- Dong J, Duan X, Feng R, et al. Diagnostic implication of fibrin degradation products and D-dimer in aortic dissection. Sci Rep 2017;7:43957. [Crossref] [PubMed]
- Song R, Xu N, Luo L, et al. Diagnostic Value of Aortic Dissection Risk Score, Coagulation Function, and Laboratory Indexes in Acute Aortic Dissection. Biomed Res Int 2022;2022:7447230. [Crossref] [PubMed]
- Tian L, Fan X, Zhu J, et al. Plasma D-dimer and in-hospital mortality in patients with Stanford type A acute aortic dissection. Blood Coagul Fibrinolysis 2014;25:161-6. [Crossref] [PubMed]
- Wen D, Du X, Dong JZ, et al. Value of D-dimer and C reactive protein in predicting inhospital death in acute aortic dissection. Heart 2013;99:1192-7. [Crossref] [PubMed]
- Xu Z, Cheng B, Fu S, et al. Coagulative biomarkers on admission to the ICU predict acute kidney injury and mortality in patients with septic shock caused by intra-abdominal infection. Infect Drug Resist 2019;12:2755-64. [Crossref] [PubMed]
- Albuquerque PLMM, da Silva GB Junior, Meneses GC, et al. Acute Kidney Injury Induced by Bothrops Venom: Insights into the Pathogenic Mechanisms. Toxins (Basel) 2019;11:148. [Crossref] [PubMed]
- Albuquerque PLMM, Paiva JHHGL, Martins AMC, et al. Clinical assessment and pathophysiology of Bothrops venom-related acute kidney injury: a scoping review. J Venom Anim Toxins Incl Trop Dis 2020;26:e20190076. [Crossref] [PubMed]
- Wang J, Yang B, Liu M, et al. Serum cystatin C is a potential predictor of short-term mortality and acute kidney injury in acute aortic dissection patients: a retrospective cohort study. J Thorac Dis 2022;14:2977-86. [Crossref] [PubMed]
- Yuan H, Lu L, Gao Z, et al. Risk factors of acute kidney injury induced by multiple wasp stings. Toxicon 2020;182:1-6. [Crossref] [PubMed]
- Chen S, Liu H, Li T, et al. Correlation analysis of coagulation dysfunction and liver damage in patients with novel coronavirus pneumonia: a single-center, retrospective, observational study. Ups J Med Sci 2020;125:293-6. [Crossref] [PubMed]
- Suzuki T, Distante A, Zizza A, et al. Diagnosis of acute aortic dissection by D-dimer: the International Registry of Acute Aortic Dissection Substudy on Biomarkers (IRAD-Bio) experience. Circulation 2009;119:2702-7. [Crossref] [PubMed]
- Khwaja A. KDIGO clinical practice guidelines for acute kidney injury. Nephron Clin Pract 2012;120:c179-84. [Crossref] [PubMed]
- An X, Guo X, Ye N, et al. Risk factors of acute kidney injury in patients with Stanford type B aortic dissection involving the renal artery who underwent thoracic endovascular aortic repair. Ren Fail 2021;43:1130-6. [Crossref] [PubMed]
- Zhang Y, Li C, Shen M, et al. Aortic aneurysm and chronic disseminated intravascular coagulation: a retrospective study of 235 patients. Front Med 2017;11:62-7. [Crossref] [PubMed]
- Vargas I, Stephenson DJ, Baldwin M, et al. Sustained local inhibition of thrombin preserves renal microarchitecture and function after onset of acute kidney injury. Nanomedicine 2021;38:102449. [Crossref] [PubMed]
- Scrascia G, Rotunno C, Simone S, et al. Acute kidney injury in high-risk cardiac surgery patients: roles of inflammation and coagulation. J Cardiovasc Med (Hagerstown) 2017;18:359-65. [Crossref] [PubMed]
(English Language Editor: J. Gray)