Decreased expression of perforin in CD8+ T lymphocytes in patients with Mycobacterium tuberculosis infection and its potential value as a marker for efficacy of treatment
Introduction
Tuberculosis (TB) remains a serious public health problem because of its high potential for person-to-person transmission. According to the annual report by World Health Organization, there were an estimated 10.4 million new (incident) TB cases worldwide, an estimated 480,000 new cases of multidrug-resistant TB (MDR-TB) and an estimated 1.4 million TB deaths in 2015 (1). The control of the global TB epidemic has been impaired by the emergence of drug- resistant forms of Mycobacterium tuberculosis, and the lack of an effective vaccine, the lack of sensitive and rapid diagnostics, especially in low-income and middle-income countries (2). It is estimated, by epidemiological reports, that one-third of the world’s population is latently infected with MTB and this is more than 30% in China. The majority of infected individuals develop a long-term protective immunity, which controls and contains MTB in a T cell-dependent manner. Development of TB disease results from interactions among the environment, the host, and the pathogen. Known risk factors include diabetes mellitus, HIV co-infection, immunodeficiency, malnutrition, overcrowding, and poverty. Immunity against MTB depends on a wide range of innate and adaptive immune responses driven mainly by macrophages and different T cell subsets.
The outcome of patients with pulmonary tuberculosis depends not only on the effectiveness of anti-TB drugs but also on the patients’ immune response against MTB. T-cell immunity is crucial for MTB infection control and both CD4+ and CD8+ T cells play important roles in host protection (3). It has been shown that CD8+ T cells also play an important role in containing tuberculosis infection because activated CD8+ T cells protect MTB-infected mice lacking CD4+ T cells (4). Direct killing of the microbes and killing of infected cells by CD8+T lymphocytes are needed for TB protection (5). Cytotoxicity elicited by CD8+ T lymphocytes involved granule exocytosis (GE) pathway, Fas–FasL pathway and proapoptotic cytokine [(interferon-γ) IFN-γ, IFN -α] production (6). IFN-γ has a vital function against MTB infection (7). Both CD4+ and CD8+ T cells can produce the essential effector cytokine IFN-γ (8). In the GE pathway, cytolytic (e.g., perforin and granzymes) and antimicrobial (e.g., granulysin) molecules are released from granules into the intercellular synapse between the cytotoxic T lymphocytes (CTL) and the infected target cell (9,10). The cytolytic molecule perforin forms pores in cellular membranes facilitating entry and endosome-mediated transportation of granzymes and/or granulysin to the intracellular compartments through a newly identified membrane-repair mechanism (11). Granzymes are a family of serine proteases found in granules of cytotoxic lymphocytes. In humans, five different granzymes (A, B, H, K, and M) have been identified, of which the expression are generally restricted to cells of the lymphoid lineage (12). Granzyme A and granzyme B are the predominant and well-studied of all the granzymes (13). Granzyme B is expressed constitutively in several cell types including CTL, natural killer (NK) cells, natural killer T (NKT) cells (14). A coordinated expression of effector functions such as cytolytic perforin and antimicrobial granulysin seems to be required for the control of human TB (9,15). Genetics studies in knockout mice have revealed that perforin and granzyme B are essential components of granules important in the GE mechanism of target cell death (16).
Early secreted antigenic target (ESAT)-6 and culture filtrate protein (CFP)-10 are antigens that are located in the ‘‘Region of deletion-1 (RD-1)” region in the Mycobacterium tuberculosis and these two proteins have been widely known as the stimulation antigens used in T-SPOT. The immunogenic functions of ESAT-6 and CFP-10 to CD4+ T lymphocytes have been well characterized. Recent studies explored their role in cytotoxicity responses in tuberculosis (4,9).
The main objective of the present study is to evaluate the cytotoxic state of CD8+ T lymphocytes during TB infection. We investigated the expression of IFN-γ, granzyme B and perforin in CD8+ T lymphocytes during the different disease phases of MTB infection and the changes before and after stimulation with ESAT-6 and CFP-10. ROC was analysed and whether these molecules can be used as markers of treatment efficacy has also been discussed.
Methods
Ethical statement
All participants were treated in accordance with the Declaration of Helsinki on the participation of human subjects in medical research. Written informed consent was obtained from all subjects and the study was approved by the Ethics Committee of Shanghai Pulmonary Hospital (Ethics Approval Number: K17-104).
Individuals
One hundred forty-eight individuals were prospectively enrolled between Aug 2015 and Apr 2016. Subjects with HIV infection, diabetes, cancer, autoimmune diseases, immunosuppressive treatment, and patients with non-tuberculous mycobacteria (NTM) were excluded from the study. Subjects were divided into three groups: (I) active PTB patients group: 58 (32 men, 26 women, age range 17–66 years) individuals whose blood was drawn prior to starting anti-TB treatment were diagnosed as active PTB. TB was diagnosed according to the criteria by Chinese Anti-tuberculosis Association. Diagnosis criteria for PTB patients were based on epidemiologic history, signs, symptoms, and chest X-ray and CT findings consistent with TB. All patients were tested for sputum Ziehl-Neelsen acid fast staining and liquid culture using BD Magit 960 system. All included patients had positive sputum smear microscopy and a positive culture. MTB was confirmed by an immunological POCT method for detection of MTB64 antigen and molecular identification of MTB using Gene Xpert and QT-PCR. Those patients with initial symptoms within 2 months and without anti-TB treatment were recruited; (II) stable PTB patients group: 52 individuals (29 men, 23 women, age range 19–70 years) were those with confirmed pulmonary TB (PTB) and treated with standard anti-TB chemotherapy and having a negative sputum smear after 2 months. Disease improvement was also shown by Chest X-ray and CT findings; (III) Healthy controls (HC) group: 38 individuals T-spot- or PPD−(20 men, 18 women, age range 21–69 years); The detailed clinical profiles of these three groups were listed in Table 1.
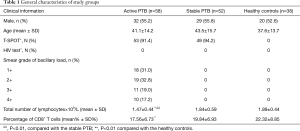
Full table
Preparation and stimulation of peripheral blood mononuclear cells (PBMC)
Heparin-treated venous blood was drawn from subjects into sterile blood collection tubes, and PBMC were isolated by density sedimentation with Ficoll-Paque Plus (GE Healthcare Life Sciences, USA). PBMC were suspended at a density of 2.5×106 viable cells/ml in complete RPMI 1640 medium (GE Healthcare Life Sciences, USA) with 10% fetal bovine serum (GE Healthcare Life Sciences, USA), penicillin (100 IU/mL) and streptomycin (100 µg/mL) (Sigma-Aldrich, USA), IL-2 (10 U/mL, Sigma-Aldrich USA). The cell suspensions were plated on 96 well plate with a quantity of 100 µL. Each sample was inoculated into two wells. One well was then stimulated with ESAT-6 and CFP-10 at a concentration as exactly as T-spot (ESAT-6 50 µL and CFP-10 50 µL as mentioned in the T-SPOT kit manual). In the blank wells, same volume culture media were added. Cells were incubated at 37 °C in a 5% CO2 humidified air atmosphere for 72 h. Brefeldin A (10 µg/mL, eBioscience, USA) was added at the latest 8-hour incubation.
T-SPOT assay
The T-SPOT assay was done according to the manufacture’s protocol (Oxford Immunote Ltd., UK).
Flow cytometry
Anti-human monoclonal antibodies CD8 (APC), IFN-γ (FITC), granzyme B (PE), perforin (PE-Cy7) and isotype antibodies were all purchased from eBioscience, USA. Cells were carefully harvested. Surface staining of CD8 antibodies (10 µL) was incubated at room temperature for 30 min in the dark. For the intracellular staining, cells were fixed for 30 min in Cytofix/Cytoperm (eBioscience, USA) at 4 °C in the dark and then washed with Perm/Wash buffer. Subsequently, the cells were re-suspended in Perm/wash buffer containing the antibodies against IFN-γ, granzyme B, and perforin. The samples were analyzed by flow cytometry with an FACSCanto II (BD Bioscience). Instruments were calibrated daily using the “7-color setup beads” calibration system (BD) to optimize the stability. The FlowJo software (Tree Star Inc, Ashland, OR, USA) was used to analyze the data. Lymphocytes were gated in the forward scatter versus side scatter dot plot. CD8+ T lymphocytes were selected and expression of IFN-γ, granzyme B, and perforin was analyzed. The results are expressed as a percentage of cells in the CD8+ T lymphocyte populations.
Statistical analysis
The statistical analysis was carried out with SPSS v.22 for Windows and GraphPad Prism version 6.0 as well as SAS™ 9.3 software (Cary, NC, USA). The mean values and SD were calculated. Mean ± Standard Deviation (SD) are shown in the figures. Differences between three independent groups were evaluated by one-way ANOVA analysis, Post Hoc Multiple Comparisons with Bonferroni or Tamhane’s T2 procedure.
A logistic regression model was used to estimate the probability of being classified into one group by combining two or more indexes. A receiver operating characteristic (ROC) curve was used to assess the diagnostic accuracy (sensitivity and specificity). The Youden index, calculated as sensitivity+specificity-1, was used for capturing the maximum vertical distance of the ROC curve and for determining cut-off points. All tests were two-sided and P value <0.05 was considered as statistical significant and the cut-off level for statistical significance for all analysis.
Results
Patients and controls characteristics
The characteristics of patients and controls included in the present study are provided in Table 1. Absolute lymphocytes number in the active PTB group was much lower than the healthy controls and the stable PTB group. Percentage of CD8+ T lymphocytes were also decreased in the active PTB than the healthy controls.
Intracellular expression of IFN-γ, granzyme B and perforin by CD8+ T lymphocyte populations
Results of flow cytometry analysis of intracellular expression of IFN-γ, granzyme B and perforin in CD8+ T lymphocytes before stimulation are shown in Figure 1. Perforin was significantly decreased in the active PTB and increased gradually as the disease ameliorated (active PTB 9.86%±3.97% vs. stable PTB 13.92%±4.34%, P=0.000). IFN-γ was most highly expressed in the active PTB and decreased as disease ameliorated (active PTB 1.76%±0.74% vs. healthy controls 0.84%±0.48%, P=0.000). Although granzyme B was most highly expressed in the active PTB, a difference of granzyme B expression was not statistically significant. Representative flow cytometry results are attached in Figure S1.
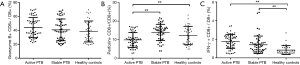
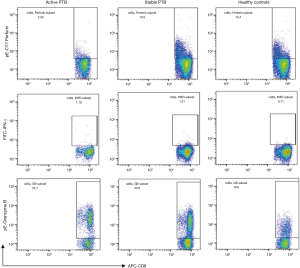
After stimulation with the combined ESAT-6 and CFP-10 peptides, perforin expression was decreased in active PTB (after stimulation 6.58%±2.95% vs. before stimulation 9.86%±3.97%, P=0.000) and stable PTB (after stimulation 10.27%±4.20% vs. before stimulation 13.92%±4.34%, P=0.000) patients, while IFN-γ was increased in active PTB (after stimulation 2.37%±1.34% vs. before stimulation 1.76%±0.74%, P=0.002) and stable PTB (after stimulation 1.92%±1.22% vs. before stimulation 1.41%±0.94%, p=0.020) patients. Expression of granzyme B was also decreased in active PTB (after stimulation 40.65%±14.00% vs. before stimulation 43.87%±14.40%, P=0.000) and stable PTB (after stimulation 38.04%±15.13% vs. before stimulation 40.64%±15.62%, P=0.004) patients. Figure 2 is the results of expression of IFN-γ, granzyme B and perforin in CD8+ T lymphocytes before and after stimulation.
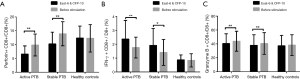
Diagnostic accuracies of perforin, IFN-γ, and granzyme B in discrimination of active PTB and stable PTB
ROC for expression of IFN-γ, granzyme B and perforin in CD8+ T lymphocytes after stimulation with ESAT-6 and CFP-10 peptides were generated by plotting sensitivity versus 1-specificity to discriminate active PTB from stable PTB (Figure 3). The cut-off value (Youden index), (area under the curve) AUC, sensitivity, specificity, PPV (positive prediction value) and NPV (negative prediction value) were showed in Table 2. Among these three markers, ROC analysis showed that changes of perforin positive rate in CD8+ T lymphocytes might be a good marker for differentiating active PTB from stable PTB. The AUC of perforin was 0.766 (P=0.000). Youden index for perforin was 7.28 (sensitivity =78.9%, specificity =72.4%). In order to evaluate using these three markers in combination, we calculated the AUC of different combination and found that the AUC for perforin + IFN-γ was 0.802, with an increased maximum sum of sensitivity (73.1%) and specificity (86.2%).
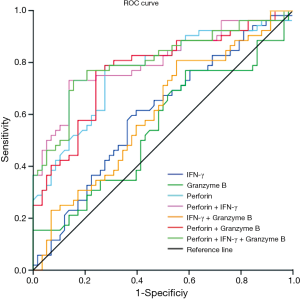
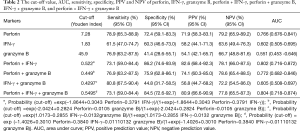
Full table
Discussion
Cytotoxicity, a mechanism for controlling pathogens essentially includes Fas/FasL interaction, lysis/apoptosis of infected cells from cytotoxic granule proteins (17). In order to understand the cytotoxicity changes in MTB, several groups have studied the role of different cytotoxic molecules, like granulysin, perforin, and granzymes in TB. A recent study suggests that granzyme B levels may not be the best proxy for CTL because a moderate decrease in granzyme B levels did not correlate with reduced in vivo killing (18). During tuberculosis, in vivo cytolytic activity is only marginally affected by the absence of perforin; however, perforin is crucial for host immunity during tuberculosis (19). In the present study, we investigated the expression of IFN-γ, granzyme B and perforin in CD8+T lymphocytes in patients with active PTB, and observed their changes after treatment. Changes of these molecules of CTL can help us to understand the immune function state of MTB patients and may be a prognosis or diagnosis marker.
IFN-γ release assays (IGRAs) have been widely used as a new tool for the immune-based diagnosis of TB. Monitoring the changes of IFN-γ using QuantiFERON-TB gold in-tube assay (QFT)(20) and an in-house ELISPOT(21) showed limited utility as a surrogate marker of treatment efficacy. In our results, we found that intracellular expression of IFN-γ in CD8+ T lymphocytes in active PTB was higher than the stable PTB, but with no statistical value (1.76%±0.74% vs. 1.41%±0.94%, P= 0.054). Our results showed that the prognosis value of IFN-γ in CD8 T lymphocytes is also limited.
The expression of granzyme A, B and K in different lymphocyte populations in PBMCs, as well as the serum levels of granzyme A and B in patients with active PTB and healthy individuals, have been reported (12). TB patients had a slightly increased expression of granzyme B in CD8+ T cells, CD4+ T cells, but not in D56+T cells, NK cells compared to controls (no statistical significance) (12,22). Our study showed a similar result that a slight increase in the expression of granzyme B in CD8+ T cells from TB patients compared with the healthy controls, but there is no statistical significance. However, contradictory results were also published, showing downregulated expression of granzyme B in CD8+ T cells from active PTB patients compared to latent TB infection and healthy controls (3).
A report from Gambia investigating intracellular perforin levels showed reduced perforin expression by CD8+ T cells in PTB patients compared to healthy individuals (23). Our study observed a similar trend. A decrease in perforin positive T cells was found in the active PTB disease (9.86%±3.97%), and this downregulation was improved as the disease improved (13.92%±4.34%). However, a contradictory result was also observed in a Mexico report, which showed that perforin expression in CD8+ T lymphocytes was higher than the healthy controls (5). These contradictory results may be produced because of different ethnicity and geographic location of patients, different antibodies used and different cell processing protocols. The expression of granzyme B and perforin in CD8+ T lymphocytes needs further study.
Changes of the intracellular expression of the granzyme B and perforin after stimulation with the combined peptides reflect the cytotoxic responses during PTB infection. Our results showed that the expression of granzyme B is significantly decreased after the stimulation with the combined ESAT-6 and CFP-10 peptides in active and stable PTB patients. Perforin was also decreased after the stimulation, which is inconsistent with the previous report(9), in which peptides selected from ESAT-6 and CDP-10 decrease the expression of perforin in CD8+ T lymphocytes. In general, CD8+ CTLs exploit the GE mechanism to destroy target cells by releasing perforin and granzymes. Our results showed that during PTB infection, cytotoxicity was impaired in the active PTB, and this may be a mechanism by which TB escapes the immunity against it. ESAT-6 and CFP-10 are not only important immunogenic proteins but also themselves maybe immunosuppressive proteins.
Recent lines of evidence have highlighted the importance of transcription factors and related pathways on cytotoxicity of CD8+ CTLs. However, the underlying transcriptional mechanisms remain elusive for the most part. Numerous transcription factors have been described to play crucial roles in cytotoxic lymphocyte differentiation, including T-bet, Notch proteins, Runt-related transcription factor 3, B lymphocyte-induced maturation protein-1, the transcriptional repressor Bcl-6, and STAT proteins (24). How the TB proteins influence those transcription factors that control the expression of cytotoxic molecules such as perforin and granzymes remains unclear. Cytokines play important role in the function of CD8+ T lymphocytes, for example, it is reported that IL-12£¨ type 1 IFN, and IL-27 were all required for efficient CD8+ T cell expansion in the lungs (18). IL-12, type I IFN, and IL-27 all promote granzyme B production following MTB infection, but no substantial loss in in vivo cytolytic activity is observed (18). The expression of multiple cytokines are affected during TB infection, including increased immunosuppressive cytokines, such as IL-35 (25). These cytokines may suppress the expression of granzyme and perforin through their interaction with the transcription factors. It is a possible pathway in revealing the TB immune escape mechanism and in modifying the suppressed immune function during the early stage of TB infection.
A study using recombinant antigens ESAT-6, GlcB and MPT-51 showed that increased/normal specific CD8+ IFN-γ and IL-10 responses (26). Our results showed that IFN-γ was increased after stimulation. These results together mean that ESAT-6 and CFP-10 are important immunogenic antigens that can stimulate IFN-γ expression not only in CD4+ T lymphocytes but also in CD8+ T lymphocytes.
The results we report here show that intracellular perforin was significantly lower in patients with active PTB than stable PTB and healthy controls. Perforin and granzyme B were downregulated after stimulation with ESAT-6 and CFP-10 peptides. Based on these data, we performed a ROC analysis (Figure 3). Significant results for AUC analysis were obtained. For scoring purposes, we chose a cut-off to maximize the sum of sensitivity and specificity. We found that the AUC of perforin after stimulation is, 0.766 (P=0.000). The value of ROC curve highlights that perforin has a high sensitivity and specificity in discriminating the active PTB and stable PTB. AUC for IFN-γ is less than perforin, it has limited value as a marker of treatment efficacy. We calculated the ROC curve for the combination of perforin and IFN-γ. AUC for the two combined markers was 0.802, which is higher than the single marker. This means combined use of the positive rate of perforin and IFN-γ in CD8+ T lymphocytes can increase the sensitivity and specificity for monitoring the treatment efficacy. Exploiting the high sensitivity and specificity of the perforin, clearly points to this molecule as a potential biomarker of PTB treatment efficacy. Moreover, we would like to stress that this is a pilot study which was performed on a relatively small number of individuals and certainly the research would benefit from larger study cohorts.
Conclusions
In conclusion, our results suggest that cytotoxicity was impaired in active PTB patients. This downregulation might be caused by the peptides of ESAT-6 and CFP-10. Changes of perforin and IFN-γ in CD8+ T lymphocytes may be a good surrogate endpoint marker of treatment efficacy.
Acknowledgements
Funding: This work was funded by Shanghai Municipal Natural Science Foundation (14ZR1434500).
Footnote
Conflicts of Interest: The authors have no conflicts of interest to declare.
Ethical Statement: All participants were treated in accordance with the Declaration of Helsinki on the participation of human subjects in medical research. Written informed consent was obtained from all subjects and the study was approved by the Ethics Committee of Shanghai Pulmonary Hospital (Ethics Approval Number: K17-104).
References
- WHO. Global tuberculosis report 2016. 2016.
- Lawn SD, Zumla AI. Tuberculosis. Lancet 2011;378:57-72. [Crossref] [PubMed]
- Silva BD, Trentini MM, da Costa AC, et al. Different phenotypes of CD8+ T cells associated with bacterial load in active tuberculosis. Immunol Lett 2014;160:23-32. [Crossref] [PubMed]
- Wang J, Santosuosso M, Ngai P, et al. Activation of CD8 T cells by mycobacterial vaccination protects against pulmonary tuberculosis in the absence of CD4 T cells. J Immunol 2004;173:4590-7. [Crossref] [PubMed]
- Sada-Ovalle I, Torre-Bouscoulet L, Valdez-Vazquez R, et al. Characterization of a cytotoxic CD57+ T cell subset from patients with pulmonary tuberculosis. Clin Immunol 2006;121:314-23. [Crossref] [PubMed]
- Russell JH, Ley TJ. Lymphocyte-mediated cytotoxicity. Annu Rev Immunol 2002;20:323-70. [Crossref] [PubMed]
- Jurado JO, Pasquinelli V, Alvarez IB, et al. IL-17 and IFN-gamma expression in lymphocytes from patients with active tuberculosis correlates with the severity of the disease. J Leukoc Biol 2012;91:991-1002. [Crossref] [PubMed]
- Bold TD, Ernst JD. CD4+ T cell-dependent IFN-gamma production by CD8+ effector T cells in Mycobacterium tuberculosis infection. J Immunol 2012;189:2530-6. [Crossref] [PubMed]
- Madhan Kumar M, Raja A. Cytotoxicity responses to selected ESAT-6 and CFP-10 peptides in tuberculosis. Cell Immunol 2010;265:146-55. [Crossref] [PubMed]
- Stinchcombe JC, Griffiths GM. Secretory mechanisms in cell-mediated cytotoxicity. Annu Rev Cell Dev Biol 2007;23:495-517. [Crossref] [PubMed]
- Keefe D, Shi L, Feske S, et al. Perforin triggers a plasma membrane-repair response that facilitates CTL induction of apoptosis. Immunity 2005;23:249-62. [Crossref] [PubMed]
- Garcia-Laorden MI, Blok DC, Kager LM, et al. Increased intra- and extracellular granzyme expression in patients with tuberculosis. Tuberculosis (Edinb) 2015;95:575-80. [Crossref] [PubMed]
- Lieberman J, Fan Z. Nuclear war: the granzyme A-bomb. Curr Opin Immunol 2003;15:553-9. [Crossref] [PubMed]
- Susanto O, Trapani JA, Brasacchio D. Controversies in granzyme biology. Tissue Antigens 2012;80:477-87. [Crossref] [PubMed]
- Temmerman ST, Place S, Debrie AS, et al. Effector functions of heparin-binding hemagglutinin-specific CD8+ T lymphocytes in latent human tuberculosis. J Infect Dis 2005;192:226-32. [Crossref] [PubMed]
- Trapani JA, Smyth MJ. Functional significance of the perforin/granzyme cell death pathway. Nat Rev Immunol 2002;2:735-47. [Crossref] [PubMed]
- Barry M, Bleackley RC. Cytotoxic T lymphocytes: all roads lead to death. Nat Rev Immunol 2002;2:401-9. [PubMed]
- Booty MG, Nunes-Alves C, Carpenter SM, et al. Multiple Inflammatory Cytokines Converge To Regulate CD8+ T Cell Expansion and Function during Tuberculosis. J Immunol 2016;196:1822-31. [Crossref] [PubMed]
- Woodworth JS, Wu Y, Behar SM. Mycobacterium tuberculosis-specific CD8+ T cells require perforin to kill target cells and provide protection in vivo. J Immunol 2008;181:8595-603. [Crossref] [PubMed]
- Adetifa IM, Ota MO, Walther B, et al. Decay kinetics of an interferon gamma release assay with anti-tuberculosis therapy in newly diagnosed tuberculosis cases. PLoS One 2010;5:e12502. [Crossref] [PubMed]
- Aiken AM, Hill PC, Fox A, et al. Reversion of the ELISPOT test after treatment in Gambian tuberculosis cases. BMC Infect Dis 2006;6:66. [Crossref] [PubMed]
- Brahmbhatt S, Black GF, Carroll NM, et al. Immune markers measured before treatment predict outcome of intensive phase tuberculosis therapy. Clin Exp Immunol 2006;146:243-52. [Crossref] [PubMed]
- Smith SM, Klein MR, Malin AS, et al. Human CD8(+) T cells specific for Mycobacterium tuberculosis secreted antigens in tuberculosis patients and healthy BCG-vaccinated controls in The Gambia. Infect Immun 2000;68:7144-8. [Crossref] [PubMed]
- Saeidi A, Buggert M, Che KF, et al. Regulation of CD8+ T-cell cytotoxicity in HIV-1 infection. Cell Immunol 2015;298:126-33. [Crossref] [PubMed]
- Dong X, Yang J. High IL-35 pleural expression in patients with tuberculous pleural effusion. Med Sci Monit 2015;21:1261-8. [Crossref] [PubMed]
- de Araujo-Filho JA, Vasconcelos AC Jr, Martins de Sousa E, et al. Cellular responses to MPT-51, GlcB and ESAT-6 among MDR-TB and active tuberculosis patients in Brazil. Tuberculosis (Edinb) 2008;88:474-81. [Crossref] [PubMed]