Predictors of high flow nasal cannula failure in immunocompromised patients with acute respiratory failure due to non-HIV pneumocystis pneumonia
Introduction
In immunocompromised patients with acute respiratory failure (ARF), invasive mechanical ventilation (MV) is the major predictor of death (1). Noninvasive ventilation (NIV), which delivers assisted ventilation without an invasive artificial airway, improved the clinical outcome of immunocompromised patients with ARF by avoiding endotracheal intubation (2,3). NIV has been considered the primary ventilation strategy in these patients, although recent studies have failed to replicate previous results (4,5).
High flow nasal cannula (HFNC) is a non-invasive oxygen device that can deliver up to 100% heated and humidified oxygen via a wide-bore nasal cannula at a maximum flow rate of 60 L/min. In recent years, the use of HFNC has increased, and an extensive body of data supports the therapeutic value of HFNC in hypoxemic ARF (6). The use of HFNC in immunocompromised patients with ARF has been evaluated recently (7-9). However, the results indicated that HFNC did not reduce intubation or mortality rates compared with conventional oxygen therapy. The poor clinical outcomes associated with the use of HFNC could be due to delayed intubation after HFNC failure (10), although data on the predictors of HFNC failure in immunocompromised patients with ARF are lacking.
Pneumocystis pneumonia (PCP) is an opportunistic infection that affects immunocompromised patients, such as those with HIV infection, solid organ transplantation, and malignancy (11). Moreover, non-HIV-related PCP patients present with a more abrupt course compared with HIV patients with PCP (12) and have a high mortality due to ARF, particularly among those requiring MV (13). To evaluate the predictors of HFNC outcome in patients with ARF due to non-HIV-related PCP, we compared the baseline characteristics, clinical outcomes, and serial changes in physiologic variables and arterial blood gas (ABG) levels between the HFNC success and HFNC failure groups.
Methods
Study design and study subjects
This retrospective study was conducted at the Asan Medical Center, a 2,680-bed university-affiliated hospital in Seoul, Korea. We consecutively reviewed the medical records of adult PCP subjects (aged ≥18 years) who were admitted to the general ward or ICU for ARF between January 2011 and September 2015. For subjects with multiple episodes of PCP, only the first episode was included. The exclusion criteria were as follows: a positive HIV antibody test, suspected but not confirmed PCP, history of PCP before the current hospital or ICU admission, mild disease that required only conventional oxygen therapy, intervention with NIV or MV as a first-line ventilation, or request of a do-not-resuscitate order. HFNC failure was defined as the eventual need for MV. The study outcomes included: mortality (14-, 28-, and 60-day), ICU-free days at day 28, and the incidence of pneumothorax. The study protocol was approved by the institutional review board of the Asan Medical Center (No. 2015-1244). The institutional review board waived the requirement for informed consent because the study was retrospective, and the subjects’ records were anonymized and de-identified before analysis.
Data collection and definitions
The collected baseline demographic and clinical characteristics included underlying disease, treatment before PCP diagnosis, vital signs, laboratory data, severity of illness, co-infection, prophylaxis for pneumocystis, and PCP treatment. The vital signs and ABG levels were determined at baseline (before HFNC application) and at 3, 6, 9, 12, 15 and 18 h after that to compare early changes in ventilation between HFNC success and HFNC failure. In our institution, an ABG analysis was conducted during HFNC therapy in all cases (<1 h after initiation) and at least every 3 h up to the first 24 h of device use. Steroid use was defined as corticosteroid administration within 1 month of infection. Other administered immunosuppressants included cyclosporine, tacrolimus, mycophenolate mofetil, tumor necrosis factor alpha blockers, and specific monoclonal antibodies. The severity of illness at the time of pneumonia diagnosis was assessed using the Acute Physiology and Chronic Health Evaluation (APACHE) II score (14) and the Sequential Organ Failure Assessment (SOFA) score (15). Co-infection was defined as microbiological evidence for bacterial, viral, or fungal infection with compatible clinical features at the time of pneumonia diagnosis. Clinical decisions concerning PCP treatment regimens were made by the attending physicians. Bronchoalveolar lavage and microbiological evaluations were conducted according to standard procedures as previously described (16).
Statistical analysis
Continuous variables were presented as median and interquartile range or as mean ± SD. Categorical variables were presented as percentages. The Mann-Whitney U test was used to compare continuous variables. The chi-square or Fisher’s exact test was used to compare categorical variables, as appropriate. The incidence of intubation during ICU stay was obtained using the Kaplan-Meier curve, with intubation as a substitute of mortality. Binary logistic regression was used to identify factors that predicted 60-day mortality and HFNC failure. Multicollinearity was prevented by controlling the variables with a high correlation with each other. Repeated measures analysis of variance was used to estimate the changes in physiologic variables and ABGs in each study group. All tests of significance were two-tailed, and P values less than 0.05 were considered significant. All analyses were conducted using SPSS software version 18.0 for Windows (SPSS Inc., Chicago, IL, USA).
Results
Baseline characteristics
Of the 185 consecutive cases evaluated during the study period, 133 were excluded for the reasons listed in Figure 1. Our study subjects consisted of 52 non-HIV-related PCP patients with moderate to severe ARF who received HFNC as a first-line ventilation. There were 23 (44%) subjects in the HFNC success group and 29 (56%) in the HFNC failure group.
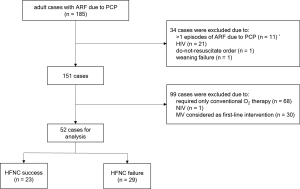
Table 1 shows the baseline characteristics of the study subjects. There were no significant differences between the groups in age, sex, body mass index, underlying disease, and use of immunosuppressive drugs before PCP diagnosis. Compared with the HFNC success group, the HFNC failure group had a significantly higher median heart rate {HFNC success group, 102 [93–116] beats/min; HFNC failure group, 117 [109–122] beats/min; P=0.01}, median alveolar-arterial PO2 difference [P(A-a)O2] {HFNC success group, 65 [48–140] mmHg; HFNC failure group, 133 [65–226] mmHg; P=0.03}, median SOFA scores {HFNC success group, 3 [3–4]; HFNC failure group, 4 [4–6]; P=0.002}, and proportion of subjects who used vasopressors (HFNC success group, 9%; HFNC failure group, 55%; P<0.001). Cytomegalovirus co-infection was observed only in the HFNC failure group. None of the evaluated subjects had recently received PCP prophylaxis. There was no intergroup difference in the time from the onset of ARF to PCP treatment, and adjunctive steroids were administered to 51 of the 52 (98%) subjects for the treatment of PCP.
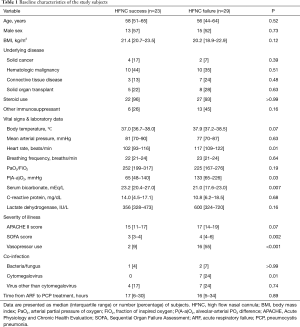
Full table
Clinical outcomes
Twelve of the 23 (52%) subjects in the HFNC success group were admitted to the ICU, whereas all subjects in the HFNC failure group were admitted to the ICU because the latter were all mechanically ventilated. Table 2 shows the clinical outcomes of the study subjects. The mortality rates (14- and 28-day) were similar between the groups, although the 60-day mortality was significantly higher in the HFNC failure group (15/29, 52%) than in the HFNC success group (3/23, 13%) (P=0.004). In the multivariate analysis, HFNC failure was significantly associated with 60-day mortality. Age and SOFA score also tended to be associated with mortality, although the association was not significant (Table 3). The HFNC failure group had fewer median ICU-free days and a higher incidence of pneumothorax compared with the HFNC success group. Figure 2 shows the Kaplan-Meier curve of the HFNC failure group, indicating that the median time from HFNC initiation to intubation was 24 [10–70] h.
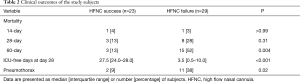
Full table
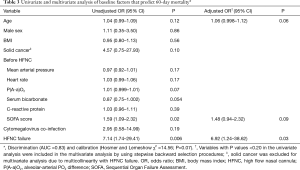
Full table
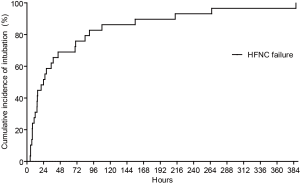
Risk factors for HFNC failure
Table 4 shows the results of univariate and multivariate analyses of baseline risk factors that predict HFNC failure. The results of multivariate analysis indicated that only SOFA score was significantly associated with HFNC failure (adjusted odds ratio, 1.74 per each score unit increase; 95% CI, 1.05–2.89; P=0.03). The serum bicarbonate level also tended to be non-significantly associated with HFNC failure (P=0.052). The HFNC failure rates according to the baseline SOFA scores are shown in Figure 3. The cutoff SOFA score that predicted HFNC failure was 4, with a sensitivity of 76% and specificity of 61%.
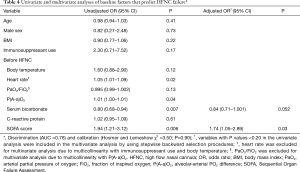
Full table
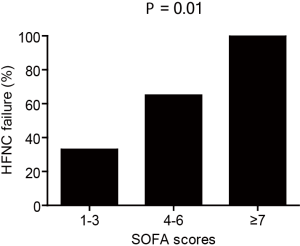
Physiologic variables and ABG response for predicting HFNC failure
The physiologic variables and ABG response during the 18-h study period are shown in Figure 4. The changes in the mean heart rate over time differed significantly between the HFNC success group and HFNC failure group. Changes in the mean breathing frequency and mean PaCO2 over time did not differ significantly between the groups. Within 6 h of HFNC initiation, the mean PaO2/FiO2 ratio increased in the HFNC success group and decreased in the HFNC failure group (P=0.03), whereas the mean P(A-a)O2 increased rapidly in the HFNC failure group during the first 18 h of the study (P=0.01).
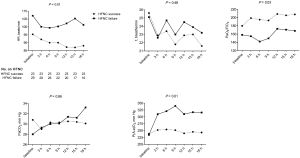
Discussion
The results of the present study indicated that higher rates of mortality and pneumothorax were observed in cases of HFNC failure, and increasing SOFA score had a positive association with HFNC failure. In the HFNC failure group, the mean heart rate remained high over time; in addition, the mean PaO2/FiO2 ratio decreased, whereas P(A-a)O2 increased within 3–6 h after HFNC initiation. These findings suggest that there is a high possibility of HFNC failure in patients with ARF caused by PCP who initially had organ dysfunction and did not show early oxygenation improvement. We limited our study to non-HIV-related PCP patients because these patients present with a more rapidly progressive course of ARF and higher mortality compared with patients with HIV-related PCP. To the best of our knowledge, this study was the first to evaluate the predictors of HFNC failure in immunocompromised patients with ARF.
Previous studies reported significant survival benefits from early NIV for ARF in cancer patients (3,17), post-operative solid organ transplant patients (2), and patients with PCP (18). However, other studies failed to confirm these results (4,5). On the other hand, in a large multicenter randomized controlled trial, HFNC has proven benefit in unselected patients with hypoxemic ARF (6), although data in immunocompromised patients are lacking. Accordingly, several studies assessed the outcomes of immunocompromised patients with ARF treated with HFNC (7-9). Compared to conventional oxygen therapy, HFNC did not reduce intubation or mortality rates. These results could be because the survival of immunocompromised patients admitted to the ICU has improved over the last 2 decades, even in patients receiving MV (1,19). In addition, delayed intubation after NIV failure (20,21) or HFNC failure (10) was associated with higher mortality. In the present study, overall 28- and 60-day mortality rates among PCP subjects with ARF who received HFNC were 21% and 35%, respectively, which are in line with the mortality rates reported in recent cohort studies involving immunocompromised patients (9,22). However, when the study subjects were divided into HFNC success and HFNC failure, 60-day mortality was significantly higher in the HFNC failure group (52%) than in the HFNC success group (13%). The results of multivariate analysis after adjusting for age, underlying disease, co-infection, and baseline severity indicated that HFNC failure was independently associated with increased mortality rates. Increased long-term mortality may be due to delayed intubation after HFNC failure and a higher rate of ventilator-associated events (e.g., pneumothorax), considering that extensive septal thickening with fibrosis and a considerably lower number of alveolar exudates is found in the late stage of severe PCP (23,24). Our study findings are relevant because the proportion of hypoxemic respiratory failure patients who use HFNC as the initial ventilation therapy is likely to increase.
Several studies have described early predictors of HFNC outcome in ARF, although these studies included patients with different types of ARF rather than patients with specific diseases (25-28). Herein we focused on immunocompromised PCP subjects with ARF and identified several clinical factors that predicted HFNC failure. The baseline heart rate, P(A-a)O2, SOFA score, and the proportion of subjects with vasopressor use were significantly higher in the HFNC failure group than in the HFNC success group. Moreover, the results of multivariate analysis indicated that the SOFA score was the only variable at baseline associated with HFNC failure. These results are consistent with findings of previous studies (26-28), which showed that the initial severity of illness and organ dysfunction might be good predictors of HFNC failure in immunocompromised patients with ARF. In addition, physiologic variables and ABG response should be determined to assess the failure of HFNC therapy. In this study, we evaluated changes within 18 h of HFNC initiation because the median time from HFNC initiation to intubation was only 24 h. As a result, the heart rate, PaO2/FiO2 ratio, and P(A-a)O2 were significantly different between the HFNC success group and HFNC failure group. P(A-a)O2 has diagnostic and prognostic value in patients with PCP (29). Our findings indicate that P(A-a)O2 may also be an accurate, early predictor of HFNC failure in PCP patients with ARF. It is of note that HFNC reduced the breathing frequency over time in the two study groups. This finding agrees with results of previous studies (26,28), suggesting that monitoring of breathing frequency alone without monitoring oxygenation may delay intubation and worsen patient outcomes.
The present study has several limitations. First, the study was single-centered, retrospective, and underpowered. Because of its retrospective nature, the criteria for starting HFNC instead of conventional oxygen therapy or NIV were absent. It can be argued that the HFNC failure group included subjects for whom it would be more appropriate to start with NIV whereas the HFNC success group could have favorable outcomes with conventional oxygen therapy alone. Moreover, the analysis of the effect of HFNC without a control group (e.g., conventional oxygen therapy or NIV) was not feasible. Lastly, the criteria for intubation (HFNC failure) were not shown. Given that the SOFA score was described as the sole predictor of HFNC failure in this study, it was possible that the attending physicians performed intubation earlier for subjects whose baseline severity was high. However, the objective of this study was to demonstrate which immunocompromised ARF patients would benefit the most from HFNC therapy, providing further evidence and rationale for the design of future randomized controlled trials. Second, our study included only a particular subgroup (PCP) of immunocompromised patients with ARF. The outcomes of HFNC may have been different in cases in which other causes of infection such as bacterial pneumonia were considered (30). Third, the type of treatment of PCP was decided by the attending physicians, and no HFNC protocol on the flow rate and FiO2 was used in this study. In addition, there was heterogeneity in the setting of HFNC therapy (general ward vs. ICU). For this reason, it was possible that the study subjects were not treated equally, and this may have affected the treatment outcomes. Fourth, the relatively long study period (>4 years) may have introduced selection and information bias. Lastly, other respiratory parameters, such as dyspnea score and thoraco-abdominal asynchrony (25), were not measured.
In conclusion, the application of HFNC as a first-line ventilation should be closely monitored in PCP patients with ARF who had organ dysfunction and did not show early oxygenation improvement. The monitoring of breathing frequency without monitoring oxygenation may contribute to HFNC failure. Further multicenter prospective studies, including patients with different ARF etiologies, are necessary to confirm our findings.
Acknowledgements
None.
Footnote
Conflicts of Interest: The authors have no conflicts of interest to declare.
Ethical Statement: The study protocol was approved by the institutional review board of the Asan Medical Center (No. 2015-1244). The institutional review board waived the requirement for informed consent because the study was retrospective, and the subjects’ records were anonymized and de-identified before analysis.
References
- Azoulay E, Mokart D, Pène F, et al. Outcomes of critically ill patients with hematologic malignancies: prospective multicenter data from France and Belgium--a groupe de recherche respiratoire en reanimation onco-hematologique study. J Clin Oncol 2013;31:2810-8. [Crossref] [PubMed]
- Antonelli M, Conti G, Bufi M, et al. Noninvasive ventilation for treatment of acute respiratory failure in patients undergoing solid organ transplantation: a randomized trial. JAMA 2000;283:235-41. [Crossref] [PubMed]
- Hilbert G, Gruson D, Vargas F, et al. Noninvasive ventilation in immunosuppressed patients with pulmonary infiltrates, fever, and acute respiratory failure. N Engl J Med 2001;344:481-7. [Crossref] [PubMed]
- Lemiale V, Resche-Rigon M, Mokart D, et al. Acute respiratory failure in patients with hematological malignancies: outcomes according to initial ventilaton strategy. A groupe de recherche respiratoire en réanimation onco-hématologique (Grrr-OH) study. Ann Intensive Care 2015;5:28. [Crossref] [PubMed]
- Lemiale V, Mokart D, Resche-Rigon M, et al. Effect of noninvasive ventilation vs oxygen therapy on mortality among immunocompromised patients with acute respiratory failure: a randomized clinical trial. JAMA 2015;314:1711-9. [Crossref] [PubMed]
- Frat JP, Thille AW, Mercat A, et al. High-flow oxygen through nasal cannula in acute hypoxemic respiratory failure. N Engl J Med 2015;372:2185-96. [Crossref] [PubMed]
- Lemiale V, Mokart D, Mayaux J, et al. The effects of a 2-h trial of high-flow oxygen by nasal cannula versus Venturi mask in immunocompromised patients with hypoxemic acute respiratory failure: a multucenter randomized trial. Crit Care 2015;19:380. [Crossref] [PubMed]
- Frat JP, Ragot S, Girault C, et al. Effect of non-invasive oxygenation strategies in immunocompromised patients with severe acute respiratory failure: a post-hoc analysis of a randomised trial. Lancet Respir Med 2016;4:646-52. [Crossref] [PubMed]
- Lemiale V, Resche-Rigon M, Mokart D, et al. High-flow nasal cannula oxygenation in immunocompromised patients with acute hypoxemic respiratory failure: a groupe de recherche respiratoire en réanimation onco-hématologique study. Crit Care Med 2017;45:e274-80. [Crossref] [PubMed]
- Kang BJ, Koh Y, Lim CM, et al. Failure of high-flow nasal cannula therapy may delay intubation and increase mortality. Intensive Care Med 2015;41:623-32. [Crossref] [PubMed]
- Thomas CF Jr, Limper AH. Pneumocystis pneumonia. N Engl J Med 2004;350:2487-98. [Crossref] [PubMed]
- Kovacs JA, Hiemenz JW, Macher AM, et al. Pneumocystis carinii pneumonia: a comparison between patients with the acquired immunodeficiency syndrome and patients with other immunodeficiencies. Ann Intern Med 1984;100:663-71. [Crossref] [PubMed]
- Monnet X, Vidal-Petiot E, Osman D, et al. Critical care management and outcome of severe Pneumocystis pneumonia in patients with and without HIV infection. Crit Care 2008;12:R28. [Crossref] [PubMed]
- Knaus WA, Draper EA, Wagner DP, et al. APACHE II: a severity of disease classification system. Crit Care Med 1985;13:818-29. [Crossref] [PubMed]
- Vincent JL, Moreno R, Takala J, et al. The SOFA (Sepsis-related Organ Failure Assessment) score to describe organ dysfunction/failure. On behalf of the Working Group on Sepsis-Related Problems of the European Society of Intensive Care Medicine. Intensive Care Med 1996;22:707-10. [Crossref] [PubMed]
- Choi SH, Hong SB, Ko GB, et al. Viral infection in patients with severe pneumonia requiring intensive care unit admission. Am J Respir Crit Care Med 2012;186:325-32. [Crossref] [PubMed]
- Azoulay E, Alberti C, Bornstain C, et al. Improved survival in cancer patients requiring mechanical ventilatory support: impact of noninvasive mechanical ventilatory support. Crit Care Med 2001;29:519-25. [Crossref] [PubMed]
- Confalonieri M, Calderini E, Terraciano S, et al. Noninvasive ventilation for treating acute respiratory failure in AIDS patients with Pneumocystis carinii pneumonia. Intensive Care Med 2002;28:1233-8. [Crossref] [PubMed]
- Azevedo LC, Caruso P, Silva UV, et al. Outcomes for patients with cancer admitted to the ICU requiring ventilatory support: results from a prospective multicenter study. Chest 2014;146:257-66. [Crossref] [PubMed]
- Moretti M, Cilione C, Tampieri A, et al. Incidence and causes of non-invasive mechanical ventilation failure after initial success. Thorax 2000;55:819-25. [Crossref] [PubMed]
- Adda M, Coquet I, Darmon M, et al. Predictors of noninvasive ventilation failure in patients with hematologic malignancy and acute respiratory failure. Crit Care Med 2008;36:2766-72. [Crossref] [PubMed]
- Coudroy R, Jamet A, Petua P, et al. High-flow nasal cannula oxygen therapy versus noninvasive ventilation in immunocompromised patients with acute respiratory failure: an observational cohort study. Ann Intensive Care 2016;6:45. [Crossref] [PubMed]
- Travis WD, Pittaluga S, Lipschik GY, et al. Atypical pathologic manifestations of Pneumocystis carinii pneumonia in the acquired immune deficiency syndrome. Review of 123 lung biopsies from 76 patients with emphasis on cysts, vascular invasion, vasculitis, and granulomas. Am J Surg Pathol 1990;14:615-25. [Crossref] [PubMed]
- Levine SJ. Pneumocystis carinii. Clin Chest Med 1996;17:665-95. [Crossref] [PubMed]
- Sztrymf B, Messika J, Bertrand F, et al. Beneficial effects of humidifed high flow nasal oxygen in critical care patients: a prospective pilot study. Intensive Care Med 2011;37:1780-6. [Crossref] [PubMed]
- Rello J, Pérez M, Roca O, et al. High-flow nasal therapy in adults with severe acute respiratory infection: a cohort study in patients with 2009 influenza A/H1N1v. J Crit Care 2012;27:434-9. [Crossref] [PubMed]
- Messika J, Ben Ahmed K, Gaudry S, et al. Use of high-flow nasal cannula oxygen therapy in subjects with ARDS: a 1-year observational study. Respir Care 2015;60:162-9. [Crossref] [PubMed]
- Roca O, de Acilu MG, Caralt B, et al. Humidified high flow nasal cannula supportive therapy improves outcomes in lung transplant recipients readmitted to the intenstive care unit because of acute respiraotry failure. Transplantation 2015;99:1092-8. [Crossref] [PubMed]
- Gagnon S, Boota AM, Fischl MA, et al. Corticosteroids as adjunctive therapy for severe Pneumocystis carinii pneumonia in the acquired immunodeficiency syndrome. A double-blind, placebo-controlled trial. N Engl J Med 1990;323:1444-50. [Crossref] [PubMed]
- Lee HY, Rhee CK, Lee JW. Feasibility of high-flow nasal cannula oxygen therapy for acute respiratory failure in patients with hematologic malignancies: a retrospective single-center study. J Crit Care 2015;30:773-7. [Crossref] [PubMed]