Effects of leflunomide on inflamation and fibrosis in bleomycine induced pulmonary fibrosis in wistar albino rats
Introduction
Pulmonary fibrosis is a rare and life-threatening disease of the lungs characterized by excessive production of fibrous connective tissues around the alveoli as a result of interstitial lung diseases. Idiopathic pulmonary fibrosis (IPF), a particularly severe form of pulmonary fibrosis with unknown etiology, has a life expectancy of 2-6 yr after diagnosis (1).
Lung fibrosis can also develop after viral infections and after exposure to radiotherapy, chemotherapeutic drugs, aerosolized environmental toxins and can also occur in a variety of clinical settings such as connective tissue diseases like rheumatoid arthritis and systemic sclerosis. Patients with pulmonary fibrosis are treated with corticosteroids together with cytotoxic agents such as azathioprine and cyclophosphamide and they have numerous side effects. The anti-fibrotic efficacy of immunosuppressive agents is limited and the results of long term survival rates are extremely poor with the current therapeutic approaches. Currently, the only effective treatment available for progressive lung fibrosis is lung transplantation. Therefore, it is needed new drugs with better efficacy and tolerability for the pulmonary fibrosis.
Leflunomide (LEF) is an immunomodulatory agent with antiproliferative activity that is approved for the treatment of rheumatoid arthritis and marketed in the US since 1998. It improves rheumatoid arthritis symptoms such as joint swelling and tenderness, slows the progression of joint damage caused by the disease. LEF [N-(4-trifluoromethylphenyl)-5-methylisoxazol-4-carboxamide] is a synthetic derivative of isoxazol and is a prodrug that is rapidly converted in the gastrointestinal tract and plasma to its active metabolite, A77 1726 (Figure 1). Using flow cytometric analysis of mitogen stimulated T cells, it was showed that A77 1726 prevented the cells from entering the DNA replication phase (S phase) of the cell cycle (2). Currently, two different specific mechanisms of action for A77 1726 have been identified: inhibition of de novo pyrimidine nucleotide biosynthesis in late G1 (growth) phase by inhibiting the dihydroorotate dehydrogenase enzyme (Figure 2) and inhibition of tyrosine kinases associated with the initial stage of signal transduction in G0 (resting) phase in higher doses. A77 1726 has been shown to suppress the activations of IL-1, TNF-α and NF-κB which are potent mediators of inflammation when stimulated by inflammatory agents (4). T cell activation is associated with pulmonary fibrosis and LEF regulates T cell progression through the cell cycle in an attempt to modulate proliferation. Unlike other proliferating cell types, lymphocytes cannot undergo cell division when the pathway for the de novo synthesis of pyrimidines is blocked. Consequently, we thought that LEF may attenuate the inflammation and fibrosis in pulmonary fibrosis.
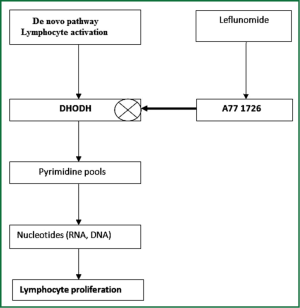
Bleomycine (BLM) induced pulmonary fibrosis is generally used as an animal model for experimental lung diseases which is developed by an initial alveolitis with increase in macrophages, granulocytes and lymphocytes. These inflammatory cells cause fibroblast proliferations and production of extracellular matrix components in the alveolar interstitial space leading to pulmonary fibrosis. We aimed in this experimental study to investigate the antiinflammatory and antifibrotic effects of LEF in BLM induced pulmonary fibrosis in rats by measurements of serum clara cell protein-16 (CC-16), thiobarbituric acid reactive substance (TBARS), superoxide dismutase (SOD) and advanced oxidation protein products (AOPP) levels and lung tissue contents of IL-6, TNF-α and NF-κB by immunhistochemical examinations.
Materials and methods
Experimental model and study groups
A total of 21 male, 6-week-old, with the weight of 250-300 g, wistar albino rats (n=7 per group; Ondokuz Mayis University Experimental Animal Center, Samsun, Turkey) were used. The animals were divided into three groups as control or sham, BLM and BLM plus LEF. In the BLM group, mice were treated with single dose of 2.5 U/kg BLM (630-107-M010, Enzo Life Sciences, USA) dissolved in sterile 0.3 mL sterile saline (0.9% NaCl) and was instilled by intratracheal injection on the day 0. The dose of BLM was selected as 2.5 U/kg which makes consistent biochemical and histological damage without mortality (5). In addition to BLM, LEF (L5025, 25 mg, Sigma-Aldrich Co., St. Louis, USA) was administrated by oral gavage, starting on day 0 and was continued until sacrifice to LEF group. The effective dose of LEF selected as 10 mg/kg/day (4). Control mice received the 0.3 mL sterile saline (0.9% NaCl) instead of BLM. To study the effect of LEF on the fibrotic phase, mice were sacrificed on day 29 after BLM instillation.
This study was approved by the institutional review board of Experimental Animal Study Ethical Comittee of Ondokuz Mayis University (Samsun, Turkey) with the decision number of 26.03.2012/26. All experimental animals were treated according to guidelines approved by the Animal Subjects Committee of the Ondokuz Mayis University.
Preparation of the samples for biochemical analysis
After sacrification of the rats, the bloods samples were collected into test tube. The whole bloods were allowed to clot at room temperature for 30 minutes. Then these samples were centrifuged at 3,000 g for 10 minutes at 4 °C. Following centrifugation, the serum was removed and transfered into a clean tube. All samples were stored at -80 °C until analysis. A day before the study all samples were dissolved at 2-8 °C.
Measurement of serum CC-16 levels
CC-16 levels were studied in line with the directions of the manufacturer company (USCN Life Science Inc., Wuhan 430056, P.R. China). The principle of the test is a sandwich enzyme immunoassay. The plate has been pre-coated with an antibody specific to CC-16. Samples are added to the wells with a biotin-conjugated antibody specific to CC-16. Next, Avidin conjugated to Horseradish Peroxidase is added to wells and incubated. After TMB substrate solution is added, the enzyme-substrate reaction is terminated by the addition of sulphuric acid solution and the color change is measured photometrically at 450 nm. The concentration of CC-16 in the samples is determined by the standard curve. The results were presented in pg/mL.
Measurement of serum TBARS levels
TBARS levels were studied in line with the directions of the manufacturer company (Catalogue No. 10009055, Cayman Chemical Company, USA). The principle of the test is based on the formation of the malondialdehyde (MDA)-TBA adduct by the reaction of MDA and TBA under high temperature (90-100 °C) and acidic conditions. Then the MDA-TBA adducts were measured colorimetrically at 530-540 nm. The results were presented in µmol/L.
Measurement of SOD levels
SOD was studied in line with the directions of the manufacturer company (Catalogue No. 706002, Cayman Chemical Company, USA). The principle of the test is based on the utilization of a tetrazolium salt for detection of superoxide radicals generated by xanthine oxidase and hypoxanthine. One unit of SOD is defined as the amount of enzyme needed to exhibit 50% dismutation of the superoxide radical. The results were presented in U/mL.
Measurement of serum AOPP levels
AOPP was studied in line with the directions of the manufacturer company (Immundiagnostik AG, Stubenwald-Allee 8a, D 64625 Bensheim, Germany). The principle of the test is based on the spectroscopic analysis of modified proteins at 340 nm. The results were presented in µmol/L.
Morphological analysis and immunohistochemical staining
The grades of pulmonary inflammation and fibrosis were analyzed by a professional histopathologist, who was blinded for groups. After taking the samples of lung tissues by appropriate techniques, they were fixed with 10% paraformaldehyde solutions in sterile phosphate-buffered saline and embedded in paraffin wax. Sections were cut at 5 μ using a microtome and deparaffinized tissue sections were subjected to Crossmon’s trichrome method and Hematoxylin-Eosin staining for histological examination.
The existence of TNF-α, IL-6 and NF-κB were investigated by using immunohistochemical streptavidin-biotin-complex method in lung tissues (6). For immunohistochemical stainings, primary antibodies of rabbit policlonal IL-6 (Abcam, AB-6672), rabbit policlonal TNF-α (Abcam, AB-9739) and mouse poliklonal NF-κB (Santa Cruz, SC-8008, CA, USA) were used. It was used LSAB + System-HRP (Santa Cruz, SC-2051, CA, USA) for detection of secondary antibodies of IL-6 and TNF-α, and Histostain Plus Rabbit Primary System (Zymed kit: 85-6743) for detection of NF-κB.
The sections were submitted to deparaffin process and permeabilized in citrate buffer (pH: 6.0) solution by microwaving at 700 watt power for proteolysis. The sections were then washed in phosphate buffer solution (PBS) and endogenous peroxidase activities were blocked by 3% H2O2 for 15 min at room temperature. After washing with PBS three times, the sections were blocked with rabbit serum for 30 min, followed by incubation with primary antibodies against the TNF-α antigen, (1:100), NF-κB antigen (1:100) and IL-6 antigen (1:500) at 4 °C, overnight. After washing with PBS, the sections were incubated in biotinylated secondary antibody for 30 min at 37 °C. Then, the sections were washed in PBS, and incubated in streptavidin-HRP complex. Antibody binding was detected with a 3,3’-diaminobenzidine kit, and the sections were counterstained with haematoxylin.
The intensity and distribution of positive staining in immunohistochemical examination was evaluated using a standard four point scorring scale for intensity, with slides being scored as negatively, weakly (+), moderately (++), and severely (+++) stained (7). Histological pictures were taken by using Nikon digital-sight imaging system attached to Nikon E-600 microscope (Nikon Corporation, Japan).
Data analysis
All data were analyzed with the SPSS 21.0 package software. The measurements obtained were expressed in mean (±) standard deviation (SD) and median (minimum-maximum) form. Due to the fact that serum CC-16 levels in sham group and serum TBARS levels in BLM groups did not comply with the normal distribution, so these measurements were compared with Mann-Whitney U tests. Other measurements were normally distributed and analyzed with Independent-Sample T tests. Statistical significance level was accepted to be P<0.05.
Results
Pulmonary fibrosis was generated as previously described, BLM-injured lungs have enhanced infiltration of inflammatory cells and the loss of normal alveolar structure compared with sham-treated mice.
Effect of LEF on CC-16, TBARS, SOD and AOPP levels
Intratracheal installation of BLM caused significant decreases in CC-16 and increases in AOPP levels compared to sham group (P=0.021 and P=0.003 respectively). There was however, no significant change in TBARS and SOD levels compared to sham group (P>0.05). LEF significantly increased the levels of CC-16 and decreased the level of AOPP (P=0.042 and P=0.003 respectively) (Figures 3,4). Details are shown in Table 1.
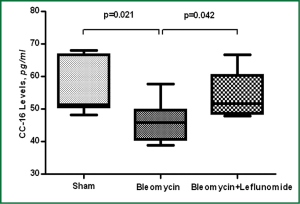
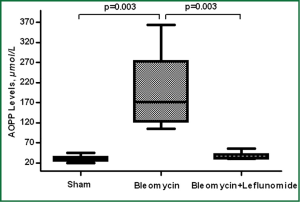
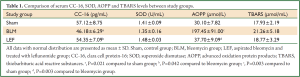
Full Table
Histopathological findings
The structures of bronchi, bronchioles and alveoles were examined and it determined some morphological differences between the groups. The intensity of degenerative changes and necrosis in the bronchial and bronchiolar walls was shown to be increased in BLM group compared to control group. Diffuse interalveolar septal thickening, accumulation of exudative fluids (edema) in alveolar space, diffuse infiltration of lymphocytes and macrophages in peribronchiolar connective tissue were the other prominent results in BLM group (Figure 5A1,A2). In LEF treated group, interalveolar septal thickening of the lung tissue was decreased compared to the BLM group. Interstitial thickening was not diffuse, and remained only in some local areas. Peribronchiolar lymphocytic infiltration was detected in connective tissues. The intensity of degenerations in bronchial and bronchiolar epithelium was decreased in LEF group compared to BLM group (Figure 5 B1,B2). Mild degenerative changes (necrosis) in bronchial walls, a mild thickening of alveolar septum and lymphocytic infiltration in interstitium and alveolar space were observed in sham group (Figure 5C1,C2).
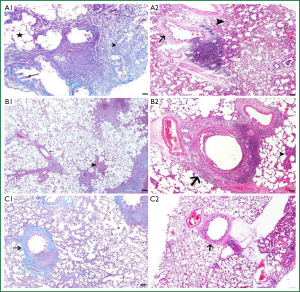
Immunohistochemical findings
IL-6 reactions
In BLM group, immunohistochemical staining of IL-6 was strongly positive in alvolar septal regions. There were strong positive IL-6 reactions in muscle cells surrounding the bronchi and bronchioles, the peripheral areas of edema, the smooth muscle cells of media layer in large blood vessels, the epithelium of the bronchi and bronchioles and alveolar macrophages (Figure 6A1). In LEF group, interalveolar septal tissue showed a quite poor reaction. A weak positive IL-6 reaction was detected in some regions especially the lymphocytic infiltration were found. Compared to BLM group a weak positive IL-6 reaction was also detected in the smooth muscle cells of peripheral airways and vessels (Figure 6A2). In sham group, IL-6 reaction was found to be negative in interalveolar septal areas. Compared to the other two groups, a mild positive IL-6 reaction was observed in peribronchial area and the blood vessel walls in sham group (Figure 6A3) (Table 2).
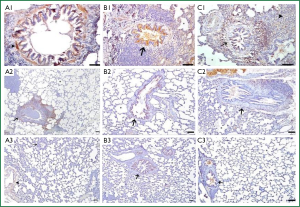
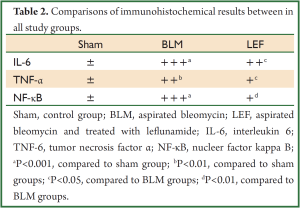
Full Table
TNF-α reactions
In BLM group, a positive immunohistochemical staining of TNF-α were observed in alveolar interseptal region, muscle cells surrounding the bronchi and bronchioles, the epithelium of the bronchi and bronchioles and alveolar macrophages (Figure 6B1). In LEF group, TNF-α reaction was not observed interalveolar septal tissue. A moderately positive reaction was observed in bronchial and bronchiolar epithelium (Figure 6B2). In sham group, the intensity and the distribution of the TNF-α reaction was similar to the LEF group (Figure 6B3).
NF-κB reactions
In BLM group, a strongly positive immunohistochemical staining of NF-κB were observed in alveolar walls, peribronchial lymphocytic infiltrated areas and alvolar interseptal regions especially in septal thickening parts (Figure 6C1). In LEF group, positive NF-κB reaction observed only in peribronchial lymphocytic infiltrated areas interalveolar septal tissue. And the reaction was extremely mild compared to BLM group (Figure 6C2). In sham group, a very weak NF-κB reaction was detected in some parts of the bronchial epithelium (Figure 6C3.
Discussion
The results of our study showed that LEF treatment may play an important role in the course of BLM induced pulmonary fibrosis with the following effects: (I) serum biochemical analysis showed that LEF increases CC-16, and decreases AOPP; (II) LEF reduces production of the inflammatory and fibrotic cytokines TNF-α, IL-6 and NF-κB; (III) LEF reduces the inflammatory cells, interalveolar septal thickening, the intensity of degenerations in bronchiolar epithelium.
Although several models of experimental pulmonary fibrosis were studied in animals, it remains unclear whether any of the experimental models exactly duplicate the IPF that is commonly seen in humans (8).These models includes drug (BLM), particulate-matter (asbestos and silica), radiation, bronchiolitis obliterans, and chronic graft versus host induced pulmonary fibrosis. However, the mouse BLM model has garnered the most attention, perhaps because it is a well-characterized and clinically relevant model of pulmonary fibrosis. In this experimental study, we used the model of BLM that was truely generated the pulmonary inflammation and fibrosis with the components of diffuse interalveolar septal thickening, accumulation of exudative fluids in alveolar space, diffuse infiltration of lymphocytes and macrophages in peribronchiolar connective tissue.
The most of the studies using the model of BLM induced fibrosis have been carried out in male rats, since the female gender is defined as a crucial factor for the development and prognosis of IPF. However, there were controversial experimental and clinical results suggesting that the estradiol and androgens play a negative role in pulmonary fibrosis (9,10). In our study histologically confirmed fibrosis was successfully achcieved in male rats with 2.5 U/kg BLM, without mortality.
LEF has been shown to prevent skin, heart, and kidney graft rejection in animal models (11,12). This drug is also effective in various experimental models of autoimmune diseases, including a spontaneous syndrome in mice that resembles human systemic lupus erythematosus, an anti-acetylcholine receptor induced model of myasthenia gravis in rats, and a T cell mediated allergic encephalomyelitis, which models human multiple sclerosis (13-15). LEF is highly effective in the treatment of animal models of both adjuvant and collagen induced arthritis and regulates lymphocyte proliferation both in vitro and in vivo studies. The gastrointestinal adverse effects such as diarrhoea, pathological changes of the small bowel and liver associated with high doses (35 mg/kg/day) in a Lewis rat model of transplantation (16). The current recommended dose of LEF is oral administration of 20 mg/kg/day in rheumatoid arthritis and if standart dosing (20 mg/kg/day) is not well tolerated, the dose may be decreased to 10 mg/kg/day (4). To our knowledge, this study is the first experimental research investigating the effects of LEF in pulmonary fibrosis and we used the dose of 10 mg/kg/day which was well tolerated by the rats with BLM induced lung injury. The optimal effective and tolerable dosage of LEF in pulmonary fibrosis is unknown and it should be investigated in different control groups.
We used the markers of TBARS, SOD and AOPP to indicate oxidative tissue damage in our study. We found a significant increase in serum AOPP levels in BLM group and due to decrease in oxidative stress its level decreased in LEF treated group. AOPP and SOD levels were not found to be different between study groups. The oxidative tissue damage could be found more severe in acute phases of BLM administration but we used only single and late phase (29th day) evaluation of serum biochemical analysis.
In recent studies, some novel markers have been found to indicate lung injury other than indicators of oxidative damage. CC-16 is produced by non-ciliated clara cells and its main function is to protect lungs against oxidative stress, inflammation and carcinogenesis (17). Its amount was found to be increased in bronchioalveolar lavage fluid of smokers and patients with lung damage (18,19) reported an increase in serum CC-16 levels in patients with sarcoidosis and IPF. We examined the serum biochemical analysis at 29th day of BLM and LEF treatments. This period represents the late phase of fibrosis. The amount of clara cells decreased in lung injury induced by BLM because of clara cell destruction and LEF as a sign of healing elevated the number of clara cells in serum.
The inflammatory mediators play a role in both the initiation and progression of pulmonary fibrosis (20). Biopsy and serum samples from patients with pulmonary fibrosis display elevated levels of TNF-α. Experimental studies also showed that overexpresses of the cytokines cause developing and progression of disease in mice (21,22). Macrophages and the other inflammatory cells produce TNF-α in the lung after exposure to particulate matters and BLM (23). Some clinical studies were recently investigated the effects of anti-TNF drugs like etanercept on IPF (24). Although inhibitors of TNF-α have shown some efficacy in pulmonary fibrosis, they might have the undesired effect such as worsening the disease (25). Therefore, it is not clear that TNF-α blockade will ameliorate pulmonary fibrosis. In this study we used the presence of TNF-α in lungs as an indicator of pulmonary inflammation and fibrosis and determined that LEF decreases the levels of TNF-α in the lungs correlating with histopathological evaluations.
There are some limitations in experimental lung studies. The pulmonary fibrosis is evaluated only by biochemical and histopathological endpoints which can not reflect the human pathological conditions (10). Recently, it has been demonstrated that efficacy of invasive pulmonary function analysis suggests that the decline in pulmonary function associated with fibrosis may not correlate with biochemical and histological endpoints in a BLM induced pulmonary fibrosis (26). Serial measurement of pulmonary function tests (particularly forced vital capacity), carbon monoxide diffusion capacity and 6 minute walk test are important predictors of survivals in patients with pulmonary fibrosis. These tests more directly correlate with survival than the severity biochemical and histopathological patterns in humans.
As a result, our findings demonstrated that LEF inhibits pulmonary inflammation, decreases IL-6, TNF-α and NF-κB production and attenuates the fibrosis in BLM induced pulmonary fibrosis. LEF holds promise as a novel drug to treat pulmonary fibrosis.
Acknowledgements
This study was funded by Ondokuz Mayis University (Samsun, Turkey) with the project number of PYO.TIP.1901.12.034.
Disclosure: The authors declare no conflict of interest.
References
- Selman M, King TE, Pardo A, et al. Idiopathic pulmonary fibrosis: prevailing and evolving hypotheses about its pathogenesis and implications for therapy. Ann Intern Med 2001;134:136-51. [PubMed]
- Cherwinski HM, McCarley D, Schatzman R, et al. The immunosuppressant leflunomide inhibits lymphocyte progression through cell cycle by a novel mechanism. J Pharmacol Exp Ther 1995;272:460-8. [PubMed]
- Herrmann ML, Schleyerbach R, Kirschbaum BJ. Leflunomide: an immunomodulatory drug for the treatment of rheumatoid arthritis and other autoimmune diseases. Immunopharmacology 2000;47:273-89. [PubMed]
- Breedveld FC, Dayer JM. Leflunomide: mode of action in the treatment of rheumatoid arthritis. Ann Rheum Dis 2000;59:841-9. [PubMed]
- Serrano-Mollar A, Closa D, Cortijo J, et al. P-selectin upregulation in bleomycin induced lung injury in rats: effect of N-acetyl-L-cysteine. Thorax 2002;57:629-34. [PubMed]
- True LD. Principles of immunohistochemistry. In: True LD. eds. Atlas of diagnostic immunohistopathologhy. New York: Gower Medical Publishing, 1990:16-22.
- Adams EJ, Gren JA, Clark AH, et al. Comparison of different scoring systems for immunohistochemical staining. J Clin Path 1999;52:75-7. [PubMed]
- Moore BB, Hogaboam CM. Murine models of pulmonary fibrosis. Am J Physiol Lung Cell Mol Physiol 2008;294:L152-60. [PubMed]
- Gharaee-Kermani M, Hatano K, Nozaki Y, et al. Gender-based differences in bleomycin-induced pulmonary fibrosis. Am J Pathol 2005;166:1593-606. [PubMed]
- Voltz JW, Card JW, Carey MA, et al. Male sex hormones exacerbate lung function impairment after bleomycin-induced pulmonary fibrosis. Am J Respir Cell Mol Biol 2008;39:45-52. [PubMed]
- Küchle CC, Thoenes GH, Langer KH, et al. Prevention of kidney and skin graft rejection in rats by leflunomide, a new immunomodulating agent. Transplant Proc 1991;23:1083-6. [PubMed]
- Williams JW, Xiao F, Foster PF, et al. Immunosuppressive effects of leflunomide in a cardiac allograft model. Transplant Proc 1993;25:745-6. [PubMed]
- Popovic S, Bartlett RR. Disease modifying activity of HWA 486 on the development of SLE in MRL/1-mice. Agents Actions 1986;19:313-4. [PubMed]
- Vidic-Dankovic B, Kosec D, Damjanovic M, et al. Leflunomide prevents the development of experimentally induced myasthenia gravis. Int J Immunopharmacol 1995;17:273-81. [PubMed]
- Bartlett RR, Anagnostopulos H, Zielinski T, et al. Effects of leflunomide on immune responses and models of inflammation. Springer Semin Immunopathol 1993;14:381-94. [PubMed]
- Chong AS, Huang W, Liu W, et al. In vivo activity of leflunomide: pharmacokinetic analyses and mechanism of immunosuppression. Transplantation 1999;68:100-9. [PubMed]
- Determann RM, Millo JL, Waddy S, et al. Plasma CC16 levels are associated with development of ALI/ARDS in patients with ventilator-associated pneumonia: a retrospective observational study. BMC Pulm Med 2009;9:49. [PubMed]
- Lindahl M, Svartz J, Tagesson C. Demonstration of different forms of the anti-inflammatory proteins lipocortin-1 and Clara cell protein-16 in human nasal and bronchoalveolar lavage fluids. Electrophoresis 1999;20:881-90. [PubMed]
- Kucejko W, Chyczewska E, Naumnik W, et al. Concentration of surfactant protein D, Clara cell protein CC-16 and IL-10 in bronchoalveolar lavage (BAL) in patients with sarcoidosis, hypersensivity pneumonitis and idiopathic pulmonary fibrosis. Folia Histochem Cytobiol 2009;47:225-30. [PubMed]
- Bringardner BD, Baran CP, Eubank TD, et al. The role of inflammation in the pathogenesis of idiopathic pulmonary fibrosis. Antioxid Redox Signal 2008;10:287-301. [PubMed]
- Miyazaki Y, Araki K, Vesin C, et al. Expression of a tumor necrosis factor-alpha transgene in murine lung causes lymphocytic and fibrosing alveolitis. A mouse model of progressive pulmonary fibrosis. J Clin Invest 1995;96:250-9. [PubMed]
- Hasegawa M, Fujimoto M, Kikuchi K, et al. Elevated serum tumor necrosis factor-alpha levels in patients with systemic sclerosis: association with pulmonary fibrosis. J Rheumatol 1997;24:663-5. [PubMed]
- Piguet PF, Vesin C. Treatment by human recombinant soluble TNF receptor of pulmonary fibrosis induced by bleomycin or silica in mice. Eur Respir J 1994;7:515-8. [PubMed]
- Raghu G, Brown KK, Costabel U, et al. Treatment of idiopathic pulmonary fibrosis with etanercept: an exploratory, placebo-controlled trial. Am J Respir Crit Care Med 2008;178:948-55. [PubMed]
- Thavarajah K, Wu P, Rhew EJ, et al. Pulmonary complications of tumor necrosis factor-targeted therapy. Respir Med 2009;103:661-9. [PubMed]
- Collard HR, King TE Jr, Bartelson BB, et al. Changes in clinical and physiologic variables predict survival in idiopathic pulmonary fibrosis. Am J Respir Crit Care Med 2003;168:538-42. [PubMed]