Right ventricular dysfunction during acute respiratory distress syndrome and veno-venous extracorporeal membrane oxygenation
Introduction
Acute respiratory distress syndrome (ARDS) is frequently complicated by pulmonary hypertension causing right ventricular (RV) failure (1). Symptoms are determined by low cardiac output and/or systemic venous congestion. Low cardiac output can result in organ failure and hemodynamic collapse. Recently, venous congestion is more and more acknowledged as an important contributor to organ failure in both heart failure and septic shock patients. RV failure results in systemic congestion with a detrimental effect particularly on kidney and liver function (2,3).
There are conflicting reports on the incidence of RV failure in ARDS, and how it affects prognosis in the current era of lung protective ventilation. Depending on definition, the incidence of RV failure in ARDS is 10–25%. Whereas several groups found RV failure in ARDS to be associated with mortality (1,4,5); other groups did not find a significant contribution to mortality (6,7). The conflicting findings can be explained by differences in study population, different definitions of RV failure, and sample size. If RV failure results in true circulatory impairment, it is a likely contributor to morbidity and mortality.
In the severe ARDS population referred for veno-venous extracorporeal membrane oxygenation (VV ECMO), pulmonary hypertension or RV failure may be present in more than half of patients and seems associated with mortality (8). This will influence patient management regarding choice of ECMO modus [VV, veno-arterial (VA) or veno-arterial and venous (VAV)], and circulatory support and ventilator settings after ECMO initiation. There is limited literature on management of RV failure during VV ECMO support. This review briefly outlines the underlying mechanisms of RV failure in ARDS and its general management. Hereafter, it will focus on how it impacts on choice of ECMO modus and patient management on ECMO support.
Causes of RV failure during ARDS
Factors directly related to the respiratory disease as well as the effects of mechanical ventilation may influence pulmonary vascular function and form a complex interplay in RV afterload. Moreover, mechanical ventilation can influence RV preload as well. Apart from RV pre- and afterload, RV function may also directly be negatively affected by sepsis (septic cardiomyopathy) and metabolic derangements like acidosis which will not be discussed in this review (9-11).
Pulmonary vascular determinants of RV afterload in ARDS
Microvascular obstruction in the lung is often present in ARDS (12,13). This obstruction can be caused by pulmonary vasoconstriction or by pulmonary capillary coagulation. Hypoxic pulmonary vasoconstriction, which is a fast acting adaptive response, decreases blood flow in poorly aerated parts of the lung, improving V/Q match. However, in a state of generalized hypoxia due to severe lung injury, as in severe pneumonia or ARDS, it contributes to increased RV afterload (14). Furthermore, in ARDS, an imbalance in mediators of vascular tone like eNOS, endothelins and prostanoids, causes a shift towards vasoconstriction (14,15).
Lung protective ventilation, using low tidal volume (Vt) and low plateau pressures (Pplateau), may result in hypercapnia in ARDS patients. Hypercapnia causes a significant increase of pulmonary arterial pressure (16), and severe hypercapnia was recently found to be an independent predictor of mortality in moderate to severe ARDS (17).
Postmortem histological studies have shown microvascular thrombi in early phases of ARDS, and loss of capillaries in later phases of ARDS (18). The links between microvascular alterations, coagulopathy and inflammation in ARDS are complex, not yet fully understood and beyond the scope of this review (19). However, microvascular thrombi and loss of capillaries will contribute to an increase of total pulmonary vascular resistance.
Effects of mechanical ventilation on RV function
There has been much debate about which component of mechanical ventilation is the most important determinant of RV afterload: Pplateau, mean airway pressure, or lung volume and Vt. The relationship between lung volume and RV impedance in normal lungs is a U-shaped curve, with the lowest RV impedance found around FRC (20). Therefore, lung overdistension by high airway pressures results in increased RV afterload. In damaged, low compliant lungs, intra-alveolar pressures will not necessarily result in overdistension and not be fully transmitted to the pleural space. Indeed, Vieillard-Baron et al. (21) demonstrated that Vt is the main determinant of RV afterload in ARDS and not airway pressure per se. Moreover, Vt and not Pplateau was significantly correlated with occurrence of RV failure in 145 ARDS patients included in the French pulmonary artery catheter study (6). Clearly, low Vt is recommended in all ARDS patients to prevent VILI, and the right ventricle will benefit from a low Vt strategy as well.
Apart from afterload, mechanical ventilation may also influence RV preload. If a high Vt or inappropriate high PEEP level results in an increase in pleural pressure, part of this pressure will be transmitted to the superior vena cava and right atrium, resulting in diminished venous return (22).
PEEP works as a two-sided sword. Too low PEEP will result in atelectasis with a detrimental effect on RV afterload. In a rat model, apart from increased afterload, atelectasis was linked to vascular leak and progressive lung injury as well (23). In contrast, using the open lung concept in adequately fluid resuscitated post cardiac surgery patients, PEEP of 14±4 cmH2O was well tolerated and did not increase RV impedance compared to conventional ventilation with a PEEP of 5 cmH2O (24). The key is that high PEEP did achieve full lung recruitment in these post cardiac surgery patients and was combined with very low Vt (4–6 mL/kg), so regional overdistension by Vt did not occur and impact of Vt on RV impedance was minimal. In ARDS, however, the disease is usually not homogenously distributed and a large part of the lung may not react to recruitment. In that situation, too high PEEP in combination with normal Vt may result in regional tidal overdistension without reversing atelectasis, increasing RV afterload. We therefore believe that increasing PEEP only benefits the patient if it is accompanied by an increase in lung compliance, as a sign of successful reversal of atelectasis not counterbalanced by regional overdistension (25).
How to diagnose
There are several modalities to assist in diagnosis and management of increased RV afterload and RV failure in patients with severe ARDS.
Echocardiography
Echocardiography is a quick noninvasive tool to monitor the right ventricle. Both transthoracic echocardiography (TTE) and transesophageal echocardiography (TEE) can be used, although TTE often delivers suboptimal images in these patients. Common echocardiographic parameters include indexes of dilatation, systolic function and RV afterload and are listed in Table 1. Full echocardiographic work-up of RV and pulmonary vascular function has been well described by Kaplan et al. (26). RV impedance is adequately reflected by pulmonary artery (PA) flow patterns. PA acceleration time correlates with mean PA pressure, with PA acceleration time >120 ms considered normal in the general population and values <100 ms indicating mean PA pressure >28 mmHg (26,27). Mean acceleration time, which is the ratio between peak PA flow and acceleration time, has been experimentally validated to correlate with RV impedance, with a decrease in mean acceleration time reflecting an increase in afterload, and can be used for follow up after adjustment of ventilator settings or other interventions (21,24,28). No normal values of the mean acceleration time are known yet. In our experience, values between 9.5 and 11 m/s2 during expiration are normal.
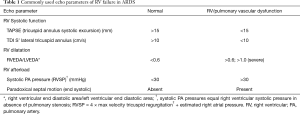
Full table
Acute cor pulmonale (ACP) is a result of (acute) increased RV afterload, and describes a situation where RV dilatation may compromise LV filling. There are several echocardiographic definitions of ACP. A widely adopted definition is the presence of both paradoxical septal movement and RV dilatation (RVEDA/LVEDA >0.6 ACP; RVEDA/LVEDA >1 severe ACP) (7). However, large interobserver variability and poor correlation with MRI derived RV volumes have been reported for RVEDA measurements and this may partly explain contradicting findings regarding the impact of ACP on prognosis (29).
Pulmonary artery catheter (PAC)
A PAC or Swan-Ganz catheter can be of great value in both diagnosis and management of ARDS patients with suspected RV failure. Apart from measuring CVP, PA and PA occlusion pressure (PAOP), it can measure the most important consequence of pulmonary vascular and RV dysfunction: reduced stroke volume and cardiac output. Continuous measurements are useful to monitor response to treatment alterations. A CVP higher than PAOP is a clear sign of RV failure, and a condition in which fluid loading can have a detrimental effect on cardiac output and venous congestion.
Pulmonary vascular resistance [(PVR) = (meanPAP − PAOP)/cardiac output] measured with Swan-Ganz catheter is not a good reflection of true RV afterload due to the high capacitance of the pulmonary vessels. Increase of flow (cardiac output) easily recruits total pulmonary vessel diameter without much increase of PA pressures (30,31). Transpulmonary pressure gradient [(TPG), mPAP − PAOP] is a flow sensitive parameter as well (32). However, an increase in TPG >12 was independently associated with mortality in ARDS patients in the FACCT trial (33).
Management of RV failure in ARDS
There are several reviews on this topic (34,35). General management include: fluid optimization, inotropes, adjustment of ventilator settings and consideration of prone positioning and inhaled Nitric Oxide (iNO).
Fluid optimization is crucial, as a failing right ventricle may initially benefit from fluid. However, fluid overloading may have a detrimental effect in these patients, worsening systemic congestion and thereby aggravating organ dysfunction. Moreover, fluid overloading promotes further RV dilatation, thereby compromising LV filling and output. Pulse pressure variation, often used as an indicator for fluid responsiveness, can be falsely elevated and may not be a good indicator of fluid responsiveness in patients with severe RV failure (36).
Vasopressors and inotropes are the second step in management of RV failure related shock. Norepinephrine increases preload by increasing systemic filling pressure, increases arterial blood pressure and increases right coronary perfusion. Dobutamine and phosphodiesterase inhibitors stimulate RV contractility and increase cardiac output. Levosimendan, a calcium sensitizer, showed beneficial effects on mean PA pressure, RV function and cardiac output in a pilot randomized trial in 35 patients with ARDS and septic shock (37).
iNO diminishes PA pressures, and positively influences V/Q matching. However, clinical trials have failed to prove a net benefit in terms of mortality or days on mechanical ventilation (38). There may be a niche indication in selected patients with severe RV failure, although meta-analyses have shown an association with kidney injury and need for renal replacement therapy after prolonged use of iNO (39,40).
As outlined in the previous chapter, Vt should be limited. PEEP and Pplateau should be on such level that unnecessary derecruitment is avoided. Prone positioning is recommended in severe ARDS to improve oxygenation, ventilation and prognosis (41). It was shown to reduce RV afterload as well, as described by Vieillard-Baron and colleagues (42).
Initiating ECMO in ARDS patients with RV failure
In patients with ARDS and RV failure, VV ECMO can generally be safely chosen as initial strategy. However, before choosing the right ECMO modus, the main origin of circulatory failure should be determined: is it cardiogenic shock due to severe RV failure, cardiogenic shock due to left heart disease or septic shock with adequate cardiac output? Of course, combinations of these factors may be present, i.e., left and right sided heart failure due to concurrent septic shock with septic cardiomyopathy in a patient with ARDS and high RV afterload. In the presence of shock due to severe LV failure, VA ECMO is indicated (Figure 1).
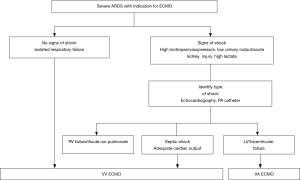
VV ECMO leads to an improvement of RV failure, as shown in a study of our group (43). In this study, 13 consecutive patients with severe respiratory failure were given a PAC before VV ECMO cannulation. Immediately after start of VV ECMO, PA pressures dropped significantly before ventilator settings were altered, followed by a slight drop in CVP and an increase in cardiac index, under stable dose of vasopressors. Both oxygenation and decarboxylation play a role here. Hypoxic pulmonary vasoconstriction reacts quickly to altered precapillary pO2 (15). In contrast, reducing ECMO sweep gas flow induced an increase of systolic PA pressures in an earlier study in ten ARDS patients as shown by Schmitt et al. (44). Another illustration of the effect of CO2-removal comes from a study on an ARDS model in pigs, in which low flow extracorporeal CO2 removal (ECCO2R) reduced PA pressures and improved RV function (45).
Since normalizing arterial pO2, pCO2 and pH may have a beneficial effect on cardiac contractility as well, we recommend starting VV ECMO for respiratory failure, even in the presence of shock due to RV failure. If shock does not improve during VV ECMO and cardiac failure is the reason for shock, then placement of an arterial cannula and switching to VAV-modus is recommended (Figure 1).
The fact that the vast majority of ARDS patient can be initiated on VV ECMO is illustrated by a study based on the ELSO registry, in which only 18% of ARDS patients with shock (one or more inotrope/vasopressor) were started on VA ECMO, and this percentage seemed dropping over the years. The rate of conversion from VV to VA ECMO in this study was low: 4.1% (46). Furthermore, a recent study of 17 ARDS patients on vasopressors qualifying for VV ECMO, reported a significant decrease in total vasopressor score after initiation of VV ECMO and no conversions to VA ECMO (47).
RV failure while on VV ECMO
If RV failure progresses during VV ECMO, one has to rethink the etiology since VV ECMO usually improves RV failure. First of all, ventilator settings can be adjusted, aiming for low Vt, far beyond 6 mL/kg, as Vt is a major determinant of RV afterload. Although there is not much evidence on ventilator settings while on VV ECMO, the Xtravent study showed that ultralow Vt of 3 mL/kg combined with ECCO2R is safe, and resulted in more ventilator free days in ARDS patients with PaO2/FiO2 ≤150 compared to conventional lung protective ventilation (48).
There are several other causes of progressive RV failure that need to be considered (Figure 2). Fluid overload can exacerbate RV failure. In general, fluid balance should be as neutral as possible in ARDS patients, with or without ECMO (49,50). Pulmonary emboli can occur, especially when anticoagulation has not been adequate or thrombus has been identified in the reinfusion cannula or the reinfusion site of the membrane. Lowering of PEEP and Vt after initiation of VV ECMO may cause derecruitment, thereby increasing RV afterload. Indeed, experts advise to maintain PEEP on a relatively high level (15 cmH2O) after starting VV ECMO in severe ARDS (51), and Schmidt et al. (52) found an association with survival comparing high PEEP during the first 3 days of ECMO versus low PEEP. Furthermore, Guervilly et al. (53) showed that prone positioning improved oxygenation in 15 ARDS patients on VV ECMO, the authors did not report RV function in this study, but it could in theory decrease RV afterload as in ARDS patients without ECMO. Finally, the original lung disease may progress and thereby increase RV afterload, as we have seen a few times in our practice.
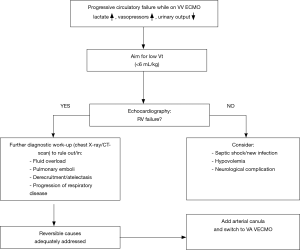
When reversible causes of progressive RV failure have been adequately addressed, and inotropes alone did not improve the situation enough, one has to consider mechanical support. Pappalardo et al. (54) reported beneficial effects of inserting an intra-aortic balloon pump (IABP) in a small group of patients with RV or biventricular failure on VV ECMO. IABP insertion resulted in lower CVP and inotrope score. Improvement of right coronary perfusion in the pressure overloaded right ventricle may be part of the explanation of its effect. The alternative or next step is switching ECMO modus. Our approach is to switch from VV- to VAVECMO by adding an arterial cannula. The venous return cannula should be partially clamped to redirect most of the flow towards the artery, the VV-flow being usually one third of the total ECMO blood flow. An alternative is to switch to VA-modus, using the subclavian artery for the arterial cannula. These two ECMO configurations prevent risk for Harlequin syndrome, which may occur with peripheral VA ECMO: supply of hypoxic blood to the upper part of the body and brain due to poor oxygenation of residual cardiac output.
A rarely practiced solution for RV failure whilst on VV ECMO is to create an ASD by percutaneous balloon atrial septostomy. This way, a right to left atrial shunt of oxygenated blood is created, unloading the right ventricle. This procedure has been described in one case report in a patient on VV ECMO with pulmonary fibrosis on the waiting list for lung transplantation (55). RV function improved and the patient could be weaned of all inotropes. The ASD closed spontaneously after transplantation. There are no reports of using this strategy in patients with ARDS or other condition awaiting recovery instead of transplantation. There is a risk of a residual ASD that needs to be closed afterwards.
Conclusions
Hypoxia, hypercarbia, and the effects of mechanical ventilation, most importantly Vt, determine RV afterload. Atelectasis can contribute as well. Although RV failure is a major concern in patients with severe ARDS, it is less a concern whilst starting ECMO support. VV ECMO has a beneficial effect on RV afterload by oxygenation, decarboxylation and normalization of pH. This improves RV function and hemodynamics. Furthermore, Vt and peak airway pressures can be reduced, additionally decreasing afterload. Therefore, if ECMO is indicated in severe ARDS, we recommend VV ECMO as initial approach. If RV failure further deteriorates while on VV ECMO, despite addressing reversible factors and treatment with inotropes, diuretics and prone positioning has been considered, then VA- or VAV-ECMO is indicated.
Acknowledgements
None.
Footnote
Conflicts of Interest: D Gommers is a member of the medical advisory board of Novalung and received travel expenses and fees for giving oral presentations from Novalung and Maquet; D Reis Miranda received speaking fees for oral presentation from Novalung and Hillrom. The other authors have no conflicts of interest to declare.
References
- Mekontso Dessap A, Boissier F, Charron C, et al. Acute cor pulmonale during protective ventilation for acute respiratory distress syndrome: prevalence, predictors, and clinical impact. Intensive Care Med 2016;42:862-70. [Crossref] [PubMed]
- Wang XT, Yao B, Liu DW, et al. Central Venous Pressure Dropped Early is Associated with Organ Function and Prognosis in Septic Shock Patients: A Retrospective Observational Study. Shock 2015;44:426-30. [Crossref] [PubMed]
- Harjola VP, Mullens W, Banaszewski M, et al. Organ dysfunction, injury and failure in acute heart failure: from pathophysiology to diagnosis and management. A review on behalf of the Acute Heart Failure Committee of the Heart Failure Association (HFA) of the European Society of Cardiology (ESC). Eur J Heart Fail 2017;19:821-36. [Crossref] [PubMed]
- Shah TG, Wadia SK, Kovach J, et al. Echocardiographic parameters of right ventricular function predict mortality in acute respiratory distress syndrome: a pilot study. Pulm Circ 2016;6:155-60. [Crossref] [PubMed]
- Boissier F, Katsahian S, Razazi K, et al. Prevalence and prognosis of cor pulmonale during protective ventilation for acute respiratory distress syndrome. Intensive Care Med 2013;39:1725-33. [Crossref] [PubMed]
- Osman D, Monnet X, Castelain V, et al. Incidence and prognostic value of right ventricular failure in acute respiratory distress syndrome. Intensive Care Med 2009;35:69-76. [Crossref] [PubMed]
- Vieillard-Baron A, Schmitt JM, Augarde R, et al. Acute cor pulmonale in acute respiratory distress syndrome submitted to protective ventilation: incidence, clinical implications, and prognosis. Crit Care Med 2001;29:1551-5. [Crossref] [PubMed]
- Lazzeri C, Cianchi G, Bonizzoli M, et al. Right ventricle dilation as a prognostic factor in refractory acute respiratory distress syndrome requiring veno-venous extracorporeal membrane oxygenation. Minerva Anestesiol 2016;82:1043-9. [PubMed]
- Parker MM, McCarthy KE, Ognibene FP, et al. Right ventricular dysfunction and dilatation, similar to left ventricular changes, characterize the cardiac depression of septic shock in humans. Chest 1990;97:126-31. [Crossref] [PubMed]
- Hunter JD, Doddi M. Sepsis and the heart. Br J Anaesth 2010;104:3-11. [Crossref] [PubMed]
- Chan CM, Klinger JR. The right ventricle in sepsis. Clin Chest Med 2008;29:661-76. ix. [Crossref] [PubMed]
- Vesconi S, Rossi GP, Pesenti A, et al. Pulmonary microthrombosis in severe adult respiratory distress syndrome. Crit Care Med 1988;16:111-3. [Crossref] [PubMed]
- Tomashefski JF Jr, Davies P, Boggis C, et al. The pulmonary vascular lesions of the adult respiratory distress syndrome. Am J Pathol 1983;112:112-26. [PubMed]
- Moloney ED, Evans TW. Pathophysiology and pharmacological treatment of pulmonary hypertension in acute respiratory distress syndrome. Eur Respir J 2003;21:720-7. [Crossref] [PubMed]
- Price LC, McAuley DF, Marino PS, et al. Pathophysiology of pulmonary hypertension in acute lung injury. Am J Physiol Lung Cell Mol Physiol 2012;302:L803-15. [Crossref] [PubMed]
- Mekontso Dessap A, Charron C, Devaquet J, et al. Impact of acute hypercapnia and augmented positive end-expiratory pressure on right ventricle function in severe acute respiratory distress syndrome. Intensive Care Med 2009;35:1850-8. [Crossref] [PubMed]
- Nin N, Muriel A, Penuelas O, et al. Severe hypercapnia and outcome of mechanically ventilated patients with moderate or severe acute respiratory distress syndrome. Intensive Care Med 2017;43:200-8. [Crossref] [PubMed]
- Bellingan GJ. The pulmonary physician in critical care * 6: The pathogenesis of ALI/ARDS. Thorax 2002;57:540-6. [Crossref] [PubMed]
- Glas GJ, Van Der Sluijs KF, Schultz MJ, et al. Bronchoalveolar hemostasis in lung injury and acute respiratory distress syndrome. J Thromb Haemost 2013;11:17-25. [Crossref] [PubMed]
- West J. Respiratory physiology. Philadelphia: Wolters Kluwer/Lippincott Williams & Wilkins, 2012.
- Vieillard-Baron A, Loubieres Y, Schmitt JM, et al. Cyclic changes in right ventricular output impedance during mechanical ventilation. J Appl Physiol (1985) 1999;87:1644-50. [Crossref] [PubMed]
- Lansdorp B, Hofhuizen C, van Lavieren M, et al. Mechanical ventilation-induced intrathoracic pressure distribution and heart-lung interactions*. Crit Care Med 2014;42:1983-90. [Crossref] [PubMed]
- Duggan M, McCaul CL, McNamara PJ, et al. Atelectasis causes vascular leak and lethal right ventricular failure in uninjured rat lungs. Am J Respir Crit Care Med 2003;167:1633-40. [Crossref] [PubMed]
- Reis Miranda D, Klompe L, Mekel J, et al. Open lung ventilation does not increase right ventricular outflow impedance: An echo-Doppler study. Crit Care Med 2006;34:2555-60. [Crossref] [PubMed]
- Amato MB, Meade MO, Slutsky AS, et al. Driving pressure and survival in the acute respiratory distress syndrome. N Engl J Med 2015;372:747-55. [Crossref] [PubMed]
- Kaplan A. Echocardiographic evaluation and monitoring of right ventricular function and pulmonary artery pressures. In: De Backer D, editor. Hemodynamic monitoring using echocardiography in the critically ill. Heidelberg: Springer-verlag Berlin, 2011:133-51.
- Rudski LG, Lai WW, Afilalo J, et al. Guidelines for the echocardiographic assessment of the right heart in adults: a report from the American Society of Echocardiography endorsed by the European Association of Echocardiography, a registered branch of the European Society of Cardiology, and the Canadian Society of Echocardiography. J Am Soc Echocardiogr 2010;23:685-713. [Crossref] [PubMed]
- Reis Miranda D, Marco Knook AH, Paalvast F, et al. Experimental validation of frequently-used echocardiographic right-ventricular impedance parameters. Minerva Anestesiol 2014;80:1169-77. [PubMed]
- Lai WW, Gauvreau K, Rivera ES, et al. Accuracy of guideline recommendations for two-dimensional quantification of the right ventricle by echocardiography. Int J Cardiovasc Imaging 2008;24:691-8. [Crossref] [PubMed]
- Versprille A. Pulmonary vascular resistance. A meaningless variable. Intensive Care Med 1984;10:51-3. [Crossref] [PubMed]
- Ryan D, Frohlich S, McLoughlin P. Pulmonary vascular dysfunction in ARDS. Ann Intensive Care 2014;4:28. [Crossref] [PubMed]
- Handoko ML, De Man FS, Oosterveer FP, et al. A critical appraisal of transpulmonary and diastolic pressure gradients. Physiol Rep 2016;4:e12910. [Crossref] [PubMed]
- Bull TM, Clark B, McFann K, et al. Pulmonary vascular dysfunction is associated with poor outcomes in patients with acute lung injury. Am J Respir Crit Care Med 2010;182:1123-8. [Crossref] [PubMed]
- Vieillard-Baron A, Matthay M, Teboul JL, et al. Experts' opinion on management of hemodynamics in ARDS patients: focus on the effects of mechanical ventilation. Intensive Care Med 2016;42:739-49. [Crossref] [PubMed]
- Repessé X, Charron C, Vieillard-Baron A. Acute respiratory distress syndrome: the heart side of the moon. Curr Opin Crit Care 2016;22:38-44. [Crossref] [PubMed]
- Mahjoub Y, Pila C, Friggeri A, et al. Assessing fluid responsiveness in critically ill patients: False-positive pulse pressure variation is detected by Doppler echocardiographic evaluation of the right ventricle. Crit Care Med 2009;37:2570-5. [Crossref] [PubMed]
- Morelli A, Teboul JL, Maggiore SM, et al. Effects of levosimendan on right ventricular afterload in patients with acute respiratory distress syndrome: a pilot study. Crit Care Med 2006;34:2287-93. [Crossref] [PubMed]
- Adhikari NK, Burns KE, Friedrich JO, et al. Effect of nitric oxide on oxygenation and mortality in acute lung injury: systematic review and meta-analysis. BMJ 2007;334:779. [Crossref] [PubMed]
- Ruan SY, Huang TM, Wu HY, et al. Inhaled nitric oxide therapy and risk of renal dysfunction: a systematic review and meta-analysis of randomized trials. Crit Care 2015;19:137. [Crossref] [PubMed]
- Afshari A, Brok J, Moller AM, et al. Inhaled nitric oxide for acute respiratory distress syndrome and acute lung injury in adults and children: a systematic review with meta-analysis and trial sequential analysis. Anesth Analg 2011;112:1411-21. [Crossref] [PubMed]
- Guérin C, Reignier J, Richard JC, et al. Prone positioning in severe acute respiratory distress syndrome. N Engl J Med 2013;368:2159-68. [Crossref] [PubMed]
- Vieillard-Baron A, Charron C, Caille V, et al. Prone positioning unloads the right ventricle in severe ARDS. Chest 2007;132:1440-6. [Crossref] [PubMed]
- Reis Miranda D, van Thiel R, Brodie D, et al. Right ventricular unloading after initiation of venovenous extracorporeal membrane oxygenation. Am J Respir Crit Care Med 2015;191:346-8. [Crossref] [PubMed]
- Schmidt M, Tachon G, Devilliers C, et al. Blood oxygenation and decarboxylation determinants during venovenous ECMO for respiratory failure in adults. Intensive Care Med 2013;39:838-46. [Crossref] [PubMed]
- Morimont P, Guiot J, Desaive T, et al. Veno-venous extracorporeal CO2 removal improves pulmonary hemodynamics in a porcine ARDS model. Acta Anaesthesiol Scand 2015;59:448-56. [Crossref] [PubMed]
- Kon ZN, Bittle GJ, Pasrija C, et al. Venovenous Versus Venoarterial Extracorporeal Membrane Oxygenation for Adult Patients With Acute Respiratory Distress Syndrome Requiring Precannulation Hemodynamic Support: A Review of the ELSO Registry. Ann Thorac Surg 2017;104:645-9. [Crossref] [PubMed]
- Gutsche JT, Mikkelsen ME, McCarthy FH, et al. Veno-Venous Extracorporeal Life Support in Hemodynamically Unstable Patients With ARDS. Anesth Analg 2017;124:846-8. [Crossref] [PubMed]
- Bein T, Weber-Carstens S, Goldmann A, et al. Lower tidal volume strategy (approximately 3 ml/kg) combined with extracorporeal CO2 removal versus 'conventional' protective ventilation (6 ml/kg) in severe ARDS: the prospective randomized Xtravent-study. Intensive Care Med 2013;39:847-56. [Crossref] [PubMed]
- National Heart, Lung, and Blood Institute Acute Respiratory Distress Syndrome (ARDS) Clinical Trials Network, Wiedemann HP, Wheeler AP, et al. Comparison of two fluid-management strategies in acute lung injury. N Engl J Med 2006;354:2564-75.
- Schmidt M, Bailey M, Kelly J, et al. Impact of fluid balance on outcome of adult patients treated with extracorporeal membrane oxygenation. Intensive Care Med 2014;40:1256-66. [Crossref] [PubMed]
- Brodie D, Bacchetta M. Extracorporeal membrane oxygenation for ARDS in adults. N Engl J Med 2011;365:1905-14. [Crossref] [PubMed]
- Schmidt M, Stewart C, Bailey M, et al. Mechanical ventilation management during extracorporeal membrane oxygenation for acute respiratory distress syndrome: a retrospective international multicenter study. Crit Care Med 2015;43:654-64. [Crossref] [PubMed]
- Guervilly C, Hraiech S, Gariboldi V, et al. Prone positioning during veno-venous extracorporeal membrane oxygenation for severe acute respiratory distress syndrome in adults. Minerva Anestesiol 2014;80:307-13. [PubMed]
- Pappalardo F, Pieri M, De Bonis M, et al. Cardiac support with IABP during venovenous ECMO for ARDS. Intensive Care Med 2013;39:1152-3. [Crossref] [PubMed]
- Kon ZN, Pasrija C, Shah A, et al. Venovenous Extracorporeal Membrane Oxygenation With Atrial Septostomy as a Bridge to Lung Transplantation. Ann Thorac Surg 2016;101:1166-9. [Crossref] [PubMed]