A novel approach for the treatment of post-pneumonectomy bronchopleural fistula by using an autologous corticocancellous bone graft
Introduction
Post-pneumonectomy bronchopleural fistula (PPBPF) is a devastating complication in thoracic surgery, with reported incidences varying from 3.1% to 20% (1-3). The diversity of incidence depends on a number of factors including malignancy or benign conditions and the extent of the resection and the resection technique used (4). When PPBPF is encountered, pneumonia with possible acute respiratory distress syndrome which usually results from the spillage of contaminated material into the contralateral healthy lung via fistula remains a major cause of mortality (2). Moreover, when PPBPF is further complicated with empyema, the condition entails repeated operative interventions, prolonged hospitalization and significantly high morbidity and mortality rates (3,5,6). Despite aggressive treatment, the reported mortality rates still range between 15% and 71% (1,2,5).
At the time of diagnosis, the choice of management of varied PPBPF depends on a number of factors consisting of the size of the fistula, occurrence of pleural space sepsis, condition of the remaining lung, and health status of the patient. Despite the widely followed protocol for treatment of PPBPF, the size of the fistula still affects the healing rates, in particular those cases larger than 3 mm in diameter (2).
At our hospital during the three years of 2008–2011, we found unsatisfactory results of treatments of patients with PPBPF. Only 29% of the cases were completely resolved of fistula, the majority of cases had adverse outcomes, with 14% in-hospital death, 43% subsequently required additional surgery to manage fistula recurrences and 14% clinically remained with a chronic empyema. Interestingly, we also found that the size of the fistula in all patients who experienced adverse outcomes exceeded 4 mm in diameter.
In an attempt to decrease the morbidity rates, length of hospital stay, and mortality rates for the patients with a larger than 4 mm of bronchopleural fistula (BPF), in June 2011, we initiated a novel treatment for these large PPBPF by using autologous corticocancellous bone grafts which have high osteogenic potential and biocompatible composite tissues that can provide viable osteoblast and bony matrix essential for the rigid healing process and we have reported the successful treatment in our first four patients (7).
Up to July 2017, the same surgeon and thoracic surgery team applied this surgical technique in 9 patients with PPBPF within 12 h of PPBPF diagnosis. The mean age of the patients of whom the fistula occurred between 2 and 18 days after the operation was 48.6 years (range, 24–68 years). The diameter of the fistula was larger than 4 mm in all cases (range, 5–14 mm) (Table 1).
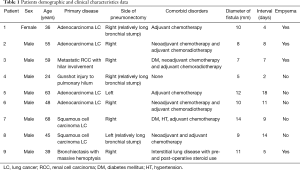
Full table
The study was approved by the Faculty of Medicine, Prince of Songkla University Ethic Committee (No. 57-0177-10-1) and written informed consents were obtained from the patients for publication of their personal data and any accompanying images.
In this article, we demonstrate the novel operative technique using autologous corticocancellous bone grafts sewed on to the inside wall of the bronchial lumen in the spiral manner from the bottom in the closing of large PPBPFs and inducing rapid healing.
Operative techniques
After pneumonectomy, when BPF was suspected as there were the presence of persistent cough, serosanguinous fluid or putrid expectoration, fever, in combination with abnormal chest radiograph that demonstrated increased air content and decreased air-fluid level within the pleural space (Figure 1A), a thoracostomy tube was immediately inserted in order to provide instant drainage of the affected pleural cavity as well as to allow an examination of pleural fluid. In all these cases, bronchial stump dehiscence was examined using flexible bronchoscopy. After the bronchoscopy confirmation of the fistula, computed tomography (CT) scan was performed in order to estimate the distance between the main carina and stump dehiscence in all patients. The empirical broad-spectrum antibiotics were given while the bacterial culture was in process. Immediately, after the diagnostic data was established, operation was performed in all patients who experienced PPBPF with the size of fistula opening larger than 4 mm in diameter.
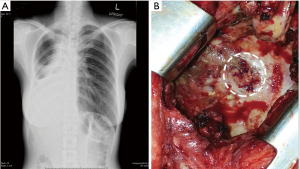
All patients underwent airway control which was enforced with a double-lumen endotracheal intubation in an attempt to prevent spillage of the infected exudate from the affected side to the contralateral healthy lung during the operation. In lateral decubitus position, a posterolateral thoracotomy was performed through the original intercostal space.
The fistulas in our patients were of various sizes and shapes such as single lumen fistula and Swiss-cheese fistula with healthy or weak or fragile residual part of the stump. All single lumen fistulas were accurately measured before debridement while each of the Swiss-cheese fistulas was cut to make one hole and accurate measurement of the actual size of the fistula was reassessed.
Measurements were preliminary taken using a bronchoscopy which provides approximate size of the fistula. Then after the fistula was cleaned and trimmed, ready for the operation, the exact size was measured using a piece of silk placed on the diameter, cut and then the cut piece was measured using a ruler.
The fistula was identified under direct vision (Figure 1B), couple with positive pressure ventilation. Prior to the bronchial repair, ipsilateral pleural cavity clearing by means of debridement of the infected or necrotic tissue and extensive irrigation with diluted povidone-iodine solution followed by normal saline was implemented. The dehiscence bronchus was meticulously dissected in a sharp-cut manner close to the bronchial wall in all patients, so as to minimize the risk of injury to the esophagus and adjacent great vessels. Care was taken to preserve the peribronchial tissue and collateral vascularization. The pathological bronchus was transected to the level where adequate blood perfusion could be seen. The resected bronchial margin and peribronchial tissue were submitted for a rapid histopathological examination to exclude any residual of the malignancy.
This present surgical method on BPF closure consists of two major stages: (I) preparing corticocancellous ossifying graft tissue; and (II) filling up the bronchus with this material using a novel suturing technique.
Preparing corticocancellous ossifying graft tissue
- The rib adjacent to the intercostal space previously approached through the pleural space on the first pneumonectomy (usually the 5th or 6th rib) was resected together with its periosteum (Figure 2A).
- After the periosteum was carefully cleaned of all muscles, the rib bone grafts were then snipped, using a pair of bone nipping rongeurs, into small (3–5 mm in diameter) pieces of composite tissues consisting of cortical bone and cancellous materials (Figure 2B). The sizes of bone pieces were snipped to fit the diameter of the bronchial lumen so that each of the bone pieces helps pack each level of the bronchus, and kept in a moist warm gauze (Figure 2C).
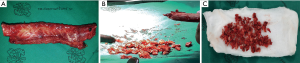
Importantly, only composite ossifying tissue which consisted of both cortical bone and cancellous bone was used as a filling material for bronchial closure.
Toward a better depiction of surgical methods, we have created simple anatomical models and drawings of the tracheobronchial tree which can be represented as a post-pneumonectomy tracheobronchial anatomy.
Filling up the bronchus
- The innermost and deepest level of an ipsilateral main bronchus was meticulously evaluated by direct visualization (Figure 3A,B) followed by the estimation of the distance between the external fistula opening and the main carina which had previously been measured from preoperative CT scan compatible images. In addition, we used a double-lumen endotracheal tube which was passed from the carina through the contralateral bronchus as a reference point of the deepest and innermost possible level of the causative bronchus for the first row of bone grafts to be sutured without harmful crossover to the orifice of the contralateral bronchus.
- A size 4-0 double-needle along with monofilament non-absorbable suture material was used in suturing. On the half-way point of the suture, several surgical knots were tied over each other to make one large knot (Figure 4A) to become the reference point for the first piece of bone graft taken down to the base of the bronchial stump.
- The needle on one end was threaded through the cancellous part of a piece of the bone grafts (Figure 4B,C). The first throw of the needle was placed at the deepest level of the bronchial lumen that was most easily viewed (Figure 4D). This first site was designated as the 12 o’clock position (Figure 5A). The bone piece was then tangentially transfixed to the bronchial lumen at this position of the inner wall (Figure 5B,C).
- The process was continued by moving both ends of the needle in the opposite direction, i.e., one end proceeded to the approximate 2 and 4 o’clock positions (Figure 6A,B) while the other proceeded to the approximate 10 and 8 o’clock positions (Figure 7).
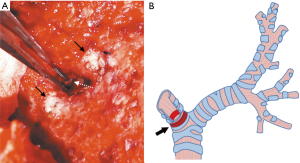
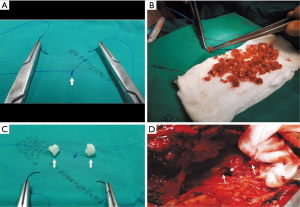
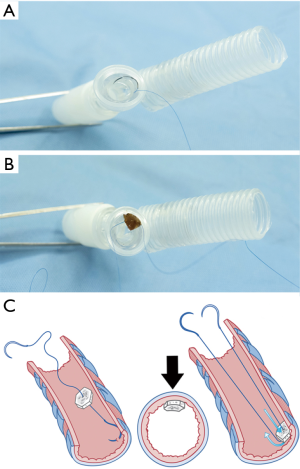
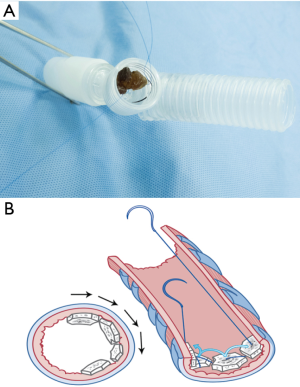
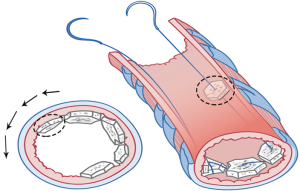
They met at the 6 o’clock position (Figure 8A,B,C) to start a new row on top of the previous one with the bone grafts sutured at approximately the same positions as those below them. The rows kept spiraling upwards (Figure 9A) with the first end of the needle always moving clockwise and the other end always counterclockwise, until the bronchial lumen was fully packed with the bone pieces (Figure 9B).
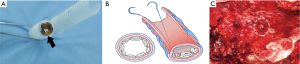
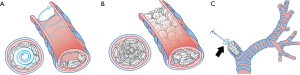
Each piece of bone grafts serves as a space filling material. Therefore, various sizes of bone graft can be selected depending on the cross-sectional area of each bronchial stump level, in order to tightly pack and close each level of the bronchus.
The bronchus was completely closed with the bone pieces to the uppermost edge. Both ends of the double-needle were secured in close proximity (Figure 9C) onto the healthy or strengthen scar tissue, and the two ends of the suturing filament were tied over a felt pledget (Figure 10A,B).
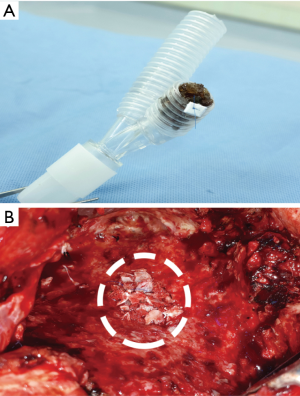
Importantly, all through the process, great care was taken not to allow any dislodgement of the bone pieces into the contralateral bronchial tree (Figure 11A,B).
The closed bronchial stump was then checked under saline covering to ensure air tightness with 40 cmH2O of sustained airway pressure.
At least 5 minutes gentle pressure was applied through a moist warm gauze against the completely filled bronchial stump. This allows appropriate clot formation around the inter-cancellous bone spaces to ensure a secure fit and air-fluid seal.
At the end of the procedure, the entire pleural cavity was irrigated with 2.0 L of normal saline. Furthermore, in the case of empyema occurrence, a bactericidal antibiotic solution was instilled directly into the pleural cavity following the saline irrigation.
A chest tube was placed posteriorly and the thoracotomy wound was closed in layers. After the patient was discharged from the operating theater, the thoracic cavity was emptied through a chest drain for 48 h, which was then removed unless a continuous air bubbling was detected in the chest drainage system or the patient experienced unusual bleeding. However, in the case of PPBPF associated with empyema, the chest drain was removed after two consecutive weekly negative cultures.
Culture-specific systemic antibiotics were administered in all patients until the removal of the drainage catheters. In fact, we did not perform a continuous intra-pleural antibiotic irrigation of the pneumonectomy space in any of the patients.
In the absence of clinical signs of fistula recurrence and infection, the patient can safely be discharged. Thereafter, subsequent follow-up chest radiography was performed at 14 and 28 days, 2 and 3 months and in combination with CT scan of the chest at 6 and 12 months after the patient was discharged from the hospital. According to our usual practice, only one bronchoscopic evaluation of bronchial healing was carried out over a period of 8 weeks after the procedure unless a recurrent fistula was suspected.
Results
There was no in-hospital mortality. A successful closure of PPBPFs with autologous corticocancellous bone graft was achieved in all cases, as evidenced by the rapid onset of symptomatic improvement and immediate cessation of air-bubbles in the chest drainage system. There was no sign of dislodgement or observable displacement of any bone grafts into the contralateral bronchial tree.
Non-hemodynamically significant postoperative arrhythmias occurred in 2 patients (22.2%) including 1 with atrial fibrillation and 1 with supraventricular tachyarrhythmia who were both successfully managed pharmacologically.
The thoracostomy tube could be removed on average of 5 days (range, 2–8 days) after the procedure (Table 2). The average duration of treatment after the procedure was 10 days (range, 5–15 days). No early empyema or fistula recurrence was observed. These results allowed all patients to be safely discharged. The position and stability of the bone grafts within the bronchus were assessed in follow-up examinations using a bronchoscopy and CT scan (Figure 12A,B,C).
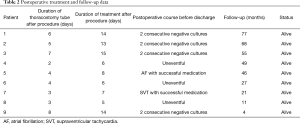
Full table
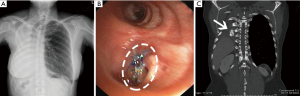
As of November 2017, permanent closure of the BPF without recurrence was achieved in all the patients with a mean follow-up time of 40 months (range, 4–77 months).
Comments
BPF is a catastrophic complication following pneumonectomy, with high morbidity and mortality rates having been reported up to 67% (2). Impaired respiratory function, contralateral lung contamination and associated empyema complicate the condition and result in poor outcomes.
Consistent with those previous reports (1,2), PPBPFs in our patients were on the right side in 7 of 9 patients (77.8%) and 88.9% (n=8) were male. Seven of the 9 patients (77.8%) harbored the perioperative risk of insufficient bronchial mucosal blood flow associated with adjuvant or neoadjuvant therapies which corresponded to the previous studies (8,9). Also, a long bronchial stump is reported as a risk factor (10), likely due to mucus accumulation leading to a higher risk of infection and impaired healing of the bronchus. We found a relatively long bronchial stump in 3 cases (33.3%), which may have had a potential role of fistula development.
Treatment priorities in PPBPF consist of airway protection with pleural drainage and early closure of the bronchial fistula. We committed to definite closure of the fistula within 12 h after diagnosis, to reduce the risk of any devastating outcomes.
Our novel approach
We initiated the use of autologous corticocancellous bone grafting from the adjacent rib to implant into the bronchial stump in order to create a completely air and water tight sealing. Generally, cortical bone grafts are used for structural support, and cancellous bone grafts for osteogenesis. This is one of the prime advantages of using bone graft, and that is why we carefully decided to use a composite of cortical and cancellous bone. Furthermore, the availability of the graft at no additional cost to the patient and the use of common and basic thoracic surgery instruments make the method attractive in our environment.
The principle involved in successful corticocancellous bone graft in healing the bronchial stump was believed to be an osteoinduction, the process of bony healing that induces undifferentiated cells to become an active osteoblast (11). Based on the biologic mechanism of corticocancellous bone, it can be explained that the fragments of corticocancellous bone, which have been implanted to the bronchus, received vascular carrier from submucosal vascular plexus. The resulted osteoinduction induced the stimulation of osteoprogenitor cells to turn into osteoblasts that induced new bony matrix formation (11), integration of small fragments of bone graft, and provided a tridimensional scaffold for ingrowth bronchial mucosa capillaries (12,13). Moreover, immunological cells, which occurred simultaneously with osteoblasts, enabled the tolerance of corticocancellous bone grafts in infected pleural space.
As blood clot formation is an immediate biological response between the adjacent corticocancellous bones, we have to induce the blood clot by stabilizing the carefully and completely packed bone grafts for a period of time through gentle pressure against the filled up bronchial stump. After that, the surface over the remodeled bronchial stump became contiguous and was sealed to the adjacent bronchial mucosa and cartilage.
Six to twelve weeks after the implantation, the healing process of corticocancellous bone provides similar bone strength when compared with normal bone. In addition, completed graft incorporation occurred in 6 months. According to our usual practice, bronchoscopic evaluation was carried out between 8 and 10 weeks after the implantation procedure, and follow-up CT scan of the chest was performed at 6 months after hospital discharge. Over the period of time, these bone grafts continued to gain strength and adequate dimensional stability as a result of biomechanical stimulation.
At the mean follow-up time of 40 months (range, 4–77 months), CT scan of the chest demonstrated complete bone graft restoration in all patients. Moreover, there was no dislodgement or encroachment of any bone piece to the contralateral bronchus at the time this study was completed.
The favorable results of the use of autologous corticocancellous bone graft in our study where neither fistula recurrence nor other specific complications occurred possibly resulted from the advantages of the material used in our method including: (I) being autologous in origin with biocompatibility properties; (II) high osteogenic potential to fuse together and provide neovascularization between grafts and bronchial mucosa in the healing process; (III) adequate mechanical strength supported by cortical bone; (IV) bone graft enabling complete filling of the bronchial stump by adjusting the size and shape of each bone chip; (V) easily accessible, easy-to-harvest and requiring no reconstruction rib.
Equally important, the meticulous suturing procedure and the double-lumen endotracheal tube ensured the correct starting position for the suture at the deepest possible point of bronchus, the tight packing of the grafts, and the prevention of graft dislodgement, hence, entailing the stability of the filler and facilitating the healing process.
Limitations
However, a negative aspect of using autologous graft in our method is that an additional operative time is required, adding the possibility of chronic donor-site pain. In fact, one patient in our study was chronically treated with morphine for chronic pain at rib harvesting site. Apart from that, the percentages of incidence of postoperative atrial fibrillation and supraventricular tachyarrhythmia were equal at 11% (n=1), which were within the range described in the literature (14).
Suggestions
These negative aspect occurrences may suggest that this technique should not potentially be used in patients with hemodynamic instability and poor nutritional status. The requirement for deep anesthesia and extended operative time increases the risks associated with general anesthesia by overwhelming hemodynamic instability and affecting bone healing process integrity. Apparently, in these high-risk surgical patients, endoscopic-based procedure should be considered as a temporary bridge in attempt to close or decrease BPF. Also, the patients should receive aggressive nutritional and rehabilitative support. After the clinical conditions stabilize, an appropriate surgical intervention may be considered.
Looking forward, we may see the greater role of bronchoscopic transplantation of bone marrow-derived mesenchymal stem cells (MSCs) therapy (15) or surgical implantation of autologous MSCs-seeded synthetic biological-absorbable graft (16) in the treatment of patients with PPBPF and especially yield promising results in patients with compromised functional and nutritional status (16).
Observations
After the operation in general, complications of bone graft dislodgement to contralateral bronchus may occur but not in our cases as we actually sewed the bone pieces on to the inside wall of the bronchial lumen. Moreover, after the operation, we monitored the signs and symptoms for contralateral inhalation, or dislodgement of bone grafts to the residual lung such as expectoration of piece of bone graft, acute onset of dyspnea and any sign of lower airway obstruction shown by chest radiograph indicating segmental or lobar atelectasis and unexplainable cause of hemoptysis. Patients with these signs and symptoms have a high index of suspicions of bone graft dislodgement to contralateral residual lung which are potential life-threatening complications.
We have a policy or guideline of practice to manage this immediately by using a bronchoscopy to assess and remove piece(s) of dislodged bone graft. After that, a double lumen intubation is inserted into the bronchus of the residual lung, and we then would consider other appropriate alternative treatments depending on the patient conditions. Looking at the healing process in all the cases, complications seem very unlikely.
To our knowledge, there are no established guidelines or expert consensus in the proper management of patients with PPBPF and no policy on how to approach this difficult problem. The treatment options available include medication therapies with pleural drainage, endoscopic-based treatments, and surgical procedures. Therefore, the current interventions appear to be complementary and treatment usually individualized. In our study, the closure of the PPBPFs suturing the bronchial stump using the autologous corticocancellous bone graft gives favorable results with no incidence of fistula recurrence. This technique can be considered a reasonable and valuable addition to the armament of therapeutic alternatives in selected patients who have an early PPBPF with a size equal to or exceeding 4 mm in diameter. Once the PPBPF has developed, early recognition, pleural space drainage, and management of infectious space or empyema are crucial. To prevent a serious complication such as aspiration pneumonia, improved nutritional status, and effective rehabilitation support are also indispensable.
Acknowledgements
The authors gratefully thank Assoc. Prof. Dr. Surasak Sangkhathat for his assistance and inspiring this work. The authors also would like to express our appreciation and sincere thanks to Asst. Prof. Dr. Monta Chatupote and Asst. Prof. Umpairat Sudhinont for their editorial and kindest help in various aspects.
Footnote
Conflicts of Interest: The authors have no conflicts of interest to declare.
Informed Consent: Written informed consent was obtained from the patient for publication of this manuscript and any accompanying images.
References
- Wright CD, Wain JC, Mathisen DJ, et al. Postpneumonectomy bronchopleural fistula after sutured bronchial closure: incidence, risk factors, and management. J Thorac Cardiovasc Surg 1996;112:1367-71. [Crossref] [PubMed]
- Hollaus PH, Lax F, el-Nashef BB, et al. Natural history of bronchopleural fistula after pneumonectomy: a review of 96 cases. Ann Thorac Surg 1997;63:1391-6; discussion 1396-7. [Crossref] [PubMed]
- Cerfolio RJ. The incidence, etiology, and prevention of postresectional bronchopleural fistula. Semin Thorac Cardiovasc Surg 2001;13:3-7. [Crossref] [PubMed]
- al-Kattan K, Cattelani L, Goldstraw P. Bronchopleural fistula after pneumonectomy for lung cancer. Eur J Cardiothorac Surg 1995;9:479-82. [Crossref] [PubMed]
- Asamura H, Naruke T, Tsuchiya R, et al. Bronchopleural fistulas associated with lung cancer operations. Univariate and multivariate analysis of risk factors, management, and outcome. J Thorac Cardiovasc Surg 1992;104:1456-64. [PubMed]
- Deschamps C, Pairolero PC, Allen MS, et al. Management of postpneumonectomy empyema and bronchopleural fistula. Chest Surg Clin N Am 1996;6:519-27. [PubMed]
- Chittithavorn V, Rergkliang C, Duangpakdee P. Successful treatment of complicated post-pneumonectomy bronchopleural fistula with autogenous corticocancellous bone graft. Interact CardioVasc Thorac Surg 2014;19 Suppl 1:S62. [Crossref]
- Yamamoto R, Tada H, Kishi A, et al. Effects of preoperative chemotherapy and radiation therapy on human bronchial blood flow. J Thorac Cardiovasc Surg 2000;119:939-45. [Crossref] [PubMed]
- Taghavi S, Marta GM, Lang G, et al. Bronchial stump coverage with a pedicled pericardial flap: an effective method for prevention of postpneumonectomy bronchopleural fistula. Ann Thorac Surg 2005;79:284-8. [Crossref] [PubMed]
- Wain JC. Management of late postpneumonectomy empyema and bronchopleural fistula. Chest Surg Clin N Am 1996;6:529-41. [PubMed]
- Klokkevold PR, Jovanovic SA. Advanced Implant Surgery and Bone Grafting Techniques. In: Newman MG, Takei HM, Carranza FA. Carranza's Clinical Periodontology (9th ed.). Philadelphia: W.B. Saunders, 2002:907-8.
- Goldberg VM, Stevenson S. Natural history of autografts and allografts. Clin Orthop Relat Res 1987.7-16. [PubMed]
- Mastrogiacomo M, Scaglione S, Martinetti R, et al. Role of scaffold internal structure on in vivo bone formation in macroporous calcium phosphate bioceramics. Biomaterials 2006;27:3230-7. [Crossref] [PubMed]
- De Decker K, Jorens PG, Van Schil P. Cardiac complications after noncardiac thoracic surgery: an evidence-based current review. Ann Thorac Surg 2003;75:1340-8. [Crossref] [PubMed]
- Petrella F, Spaggiari L, Acocella F, et al. Airway fistula closure after stem-cell infusion. N Engl J Med 2015;372:96-7. [Crossref] [PubMed]
- Aho JM, Dietz AB, Radel DJ, et al. Closure of a Recurrent Bronchopleural Fistula Using a Matrix Seeded With Patient-Derived Mesenchymal Stem Cells. Stem Cells Transl Med 2016;5:1375-9. [Crossref] [PubMed]