3D printing in adult cardiovascular surgery and interventions: a systematic review
Introduction
Three-dimensional (3D) printing is a fabrication technique used to transform digital information into physical models and has been demonstrated to have utility in multiple clinical scenarios in recent decades (1). 3D printing technology has been rapidly applied in the field of medical in recent years. In cardiovascular surgery, 3D printing can provide improved visualization of the anatomical details and guide precision operations, so the most widely reported applications have been for congenital heart diseases (2). With increasing aging and complexity of cardiac conditions, current surgical or transcatheter procedures require advanced peri-procedural imaging and individualized treatment. 3D printing may to prepare for well-tolerated treatment and provide substantial information on the accuracy of surgical reconstruction in complex cardiovascular anatomy (3). Although the use of 3D printing in cardiovascular surgery is still a relatively new development, the applications for adult cardiac and vascular surgery or catheter-based interventions are also emerging, and contemporary reviews and reports are warranted. In this review, we examine the current clinical applications of 3D printing in adult cardiovascular surgery and transcatheter interventions. We also present the clinical medical simulation of physiological or pathology conducted with 3D printing in this fields. We present the following article in accordance with the PRISMA reporting checklist (available at http://dx.doi.org/10.21037/jtd-20-455).
Methods
This systematic review was performed and reported in line with the PRISMA statement (4). We searched the PubMed and MEDLINE databases up to the end of June 2019 using medical subject headings and text words supplemented by scanning the bibliographies of the recovered articles. We combined “Three-dimensional printing”, “3D printer”, “3D printing”, “3D printed”, “rapid prototyping model”, “cardiac”, “cardiovascular”, “heart surgery”, and “adult” using the Boolean operator “AND”. The results were limited to articles written in English. Two separate researchers (CW and LZ) analyzed the data set to ensure accuracy and to identify all available studies. The details of each publication were checked to avoid duplicates. Any differences were resolved by consensus.
Inclusion criteria
All cases reported the application of 3D printing for cardiovascular surgery and interventions in the adult. To review the current extended utility of 3D printing in adult cardiovascular surgery, the articles about applications of 3D printing in the clinical medical simulator and training fields were included. Only articles published in English were included.
Exclusion criteria
Papers or cases reporting on the utility of 3D printing for congenital heart diseases, technology or basic sciences were excluded. Studies based on conference abstracts, editorials or review articles were also excluded.
Data extraction
We collected the data on the first author, published year, clinical scenarios, number of patients, age, sex, study design, clinical application and imaging modality. The study purposes and main study findings were also collected. We also recorded the outcomes of patients who were treated with 3D printing technology during the perioperative period, including morbidity, mortality, and follow-up.
Statistical analysis
Data collected were organized on an Apple Numbers (version 6.6.2) spreadsheet. Descriptive statistics were used to describe the demographic and continuous data (e.g., mean ± standard deviation). Dichotomous variables were expressed as numbers with percentages.
Results
The literature search yielded 215 publications in PubMed and MEDLINE databases. Of these, a total of 43 publications focused on 3D printing for adult cardiovascular surgery, intervention and clinical medical training were identified from the literature and included in the analysis. The publications spanned a period ranging from 2008 to 2019. In some instances, two papers were published by the same author, and only the most recent manuscript was included in the analysis. Figure 1 shows the results of the literature search according to the PRISMA.
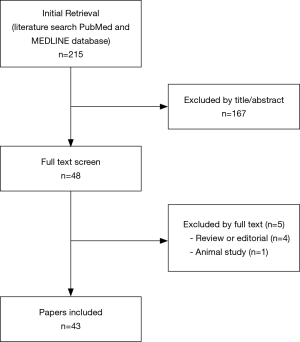
Characteristics of 3D printing in cardiovascular surgery and transcatheter interventions
Of these 43 publications, 35 were based on the clinical application of 3D printing for adult cardiovascular surgery and transcatheter interventions, and the other 8 (5-12) papers were based on physiological or pathology simulations and clinical training for cardiovascular surgery. Most studies (n=34, 97.1%) on the clinical utility of 3D printing for adult heart surgery or intervention procedures were carried out starting in 2014, and increased year by year (Figure 2). Of the 35 papers about clinical applications, 26 (74.3%) were case reports, 4 were cross-sectional studies, and 4 were retrospective studies. One study was a mix of retrospective and prospective analyses (13).
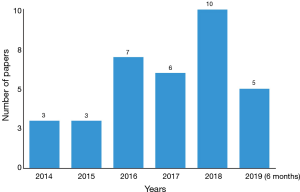
A total of 296 patients were described, with an average age of 65.4±14.2 years. In the group, 160 patients were men, and 115 patients were women. Seven reports (21 patients) did not provide the patients’ sex. Only 4 case reports (including 23 patients) provided follow-up data (6–12 months). In this research, there were no series that aimed to determine the effects of 3D printing on morbidity and mortality. Regarding the patients’ risk scores, none of the articles reported EuroSCORE data or the Society of Thoracic Surgeons (STS) score. They all reported that the included patients had generic complex anatomy. All reports recognized that a unique patient-specific 3D printing model before surgery or intervention could intuitively present complex anatomy, assist in preoperative surgical decision-making and provide intraoperative surgical guidance. Table 1 summarizes the characteristics of the eligible studies in this review.
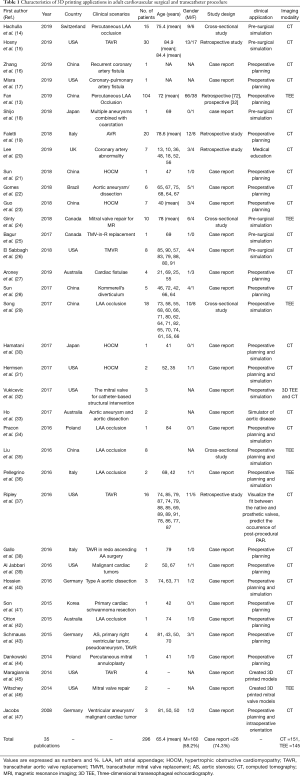
Full table
The clinical applications of 3D printing in cardiovascular surgery and transcatheter intervention
In this review, ten clinical scenarios were included: left atrial appendage occlusion (LAAO), transcatheter aortic valve replacement (TAVR), mitral valve disease treated with catheter-based intervention or open repair, aortic valve replacement, coronary artery abnormality (fistula), aortic aneurysm and dissection, hypertrophic obstructive cardiomyopathy (HOCM), Kommerell’s diverticulum, primary cardiac tumor, and ventricular aneurysm. Table 2 shows the analysis of clinical scenarios that indicated the utility of 3D printing in adult cardiovascular surgery and transcatheter procedures.
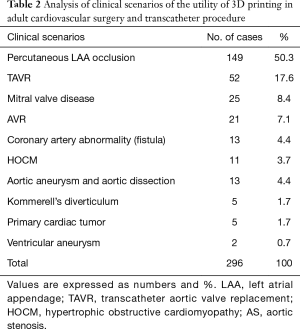
Full table
Peri-interventional 3D printing was applied in the treatment of the majority of adult heart diseases, including LAAO, TAVR, and mitral valve interventions. Of all patients, 50.3% underwent LAAO, and 17.6% underwent TAVR. The treatments for other diseases were rarely reported.
Of these 35 studies, 30 reported on 3D printing for preoperative planning or simulations and showed that models were very helpful for preoperative planning and orientation, as well as to simulate procedures due to the exact and life-like illustration of the cardiovascular anatomy. Three studies (19,45,46) reported on the feasibility and diagnostic accuracy of creating 3D-printed heart models. One study reported on visualizing the fit between the native and prosthetic valves and predicting the occurrence of post-procedural paravalvular aortic regurgitation (PAR) with preoperative 3D printing (37).
There are two main imaging techniques that are used to generate 3D-printed heart models, CT and echocardiography. Twenty-eight studies (151 cases) used CT datasets as the data source for 3D printing; 6 studies (145 cases) used transesophageal echocardiography (TEE) datasets, while only 1 study used a combination of CT and echocardiographic datasets, and no 3D-printed models were created from MRI. This is different from the 3D-printed models created for congenital heart diseases, which MRI is the most commonly used imaging modality (2). In the cohort that used TEE datasets, LAAO was the most common treatment method (88.6%).
The utility of 3D printing for clinical medical simulators or training
There were 8 papers focused on the application of 3D printing for clinical medical simulator and training in adult cardiac and vascular surgery. Six studies successfully created aortic valves, mitral valves, aortic roots or heart simulators for surgical training or improved the understanding of disease among surgical trainees (7-12). Two studies demonstrated the utilization of 3D-printed models for in vitro physiological simulations to better replicate and understand the functional physiology of the coronary artery or mitral valve (5,6). All studies assessed the usefulness of 3D-printed models in medical teaching, surgical trainee training, and patient-doctor communication and the most recent applications of 3D models in surgical planning and simulation.
Discussion
Identifying and visualizing anatomy is critical for any surgical intervention, and digital and physical preoperative anatomical assessments are an important adjunct to surgical planning (48). Advanced surgical and catheter-based procedures for structural heart disease require precise preprocedural planning and intraoperative orientation. In the past ten years, 3D printing has seen a rapid increase in adoption rate for adult cardiovascular surgery and interventions, and this technology offers unique advantages for complex anatomy, morphology and pathology, with great potential for tailored diagnostic assessments and preoperative planning.
The clinical application of 3D printing in adult heart surgery and interventions
The purpose of 3D printing for adult heart disease can be summarized into three main fields: preoperative planning or simulation, medical teaching and clinical consultations. Given the data analysis in this review, preoperative planning or simulation was the major clinical utility of 3D printing.
Perioperative 3D printing
According to the data of this research, 3D printing in adult cardiac surgery was primarily used for complicated lesions, including coronary artery abnormalities, HOCM, primary cardiac tumors and ventricular aneurysms. 3D-printed models have been reported to minimize the surgical risks and optimize surgical planning and intraoperative decision-making (16,17,21,28,47). In great vessel surgery, 3D printing can be used to accurately replicate the anatomical details of complex congenital malformations of the aorta and aortic aneurysm and dissection, including the intimal flap (33,40). 3D valve models of AS and mitral valve pathology are useful for preoperative patient-specific hemodynamic testing and are potentially helpful in improving the ability of inexperienced surgeons and the efficiency of experienced surgeons performing complex valve repairs (24).
Peri-interventional 3D printing
The benefits of peri-interventional 3D printing have been shown for percutaneous LAAO, TAVR, and mitral valve interventions (13-15,25,26,29,32,34-38,42,45). LAAO is highly associated with risks for occlusion-related complications, such as interatrial communication and device malposition, especially in LAA with complex morphology. Song et al. (29) reported that the consistency of the morphological classifications of the LAA based on 3D models and cardiac computed tomography was 0.92 (P<0.01). 3D models of the LAA prior to the procedure are helpful in finding the correct position and device size and type.
Pre-TAVR 3D printing improves the understanding of the interaction between the prosthetic valve and the patient’s anatomy in advance of surgical deployment. A retrospective study (37) showed that an examination of the fit of valves with patient-specific aortic root models correctly predicted PAR in 6 of 9 patients (6 true-positives, 3 false-negatives) and the absence of PAR in 5 of 7 patients (5 true-negatives, 2 false-positives); additionally, the finished pre-TAVR 3D-printed model could be used to visualize the fit between the native aortic valve complex and TAVR prosthetic valves and thus predict the occurrence of post-procedural PAR. For complex anatomy, e.g., extreme porcelain aorta, peri-valvular calcifications, and valve-in-valve implantation, pre-TAVR 3D printing may help achieve optimal long-term results after TAVR (43).
Transcatheter mitral valve replacement (TMVR) is an evolving therapeutic option to treat patients with severe MAC who are not surgical candidates. Guerrero et al. demonstrated that TMVR in patients with severe MAC was feasible but associated with a mortality of up to 30%, LVOT obstruction rate of 3%, and stroke rate of 3% (49). El Sabbagh et al. (26) reported that 3D printing for TMVR was feasible and could simulate valve sizing, apposition, expansion, PVL, and LVOT obstruction risk as well as other potential pitfalls, such as extra-annular calcifications.
Medical teaching and training
After almost three decades of experience with medical 3D printing, this technology is now being used to create models, even those that are very complex, as needed in cardiovascular surgery (50). 3D printing has also been shown to be a novel teaching approach in medical education to help students, cardiologists, and surgeons-in-training gain a better understanding of pathologies and surgical procedures. For young surgeons with little experience, surgical simulations with patient-specific 3D-printed structures could flatten the learning curve for difficult procedures and improve preprocedural planning (31). Moreover, the patients and their families will also be able to understand the pathology and surgical treatment more comprehensively with 3D-printed models.
3D printing and clinical outcomes
In this review, none of the research reported clinical outcomes, e.g., mortality and morbidity. The clinical benefits of 3D printing, including improved safety and better long-term results, have not been reported. There are no controlled trials that show outcome data. Owing to increasing the accuracy of preoperative planning, it maybe has the potential to decrease morbidity or mortality and improve outcomes in patients with complex heart disease as this technology becomes more widespread. However, clinical control trials with large samples are needed.
3D printing and diagnostic tools
Traditional imaging, e.g., CT, MRI and echocardiography, does not always provide all of the information required for operative planning, especially in complex cases. A 3D-printed model of the heart can provide a more complete understanding of the intracardiac anatomy to better diagnose heart disease. However, the time required for 3D printing depends on the source of the DICOM data (CT, MRI, or echocardiography), the printing technology, the size and complexity of the patient-specific model. With current 3D printing technology, 3D printing typically takes 3–10 hours to build a single piece of the heart model (51). An aortic valve requires approximately 1.5 hours, a whole heart or the aortic wall from the aortic root to the level of the mid-descending aorta requires 6–8 hours (52). A 3D printing model also requires post-processing. The complex segmentation of multiple anatomic elements might take up to 12 hours to print, which may not be suitable for clinical application in an emergency, such as acute aortic dissection (40,53). Another limitation of 3D printing for diagnosis is the possibility of errors during segmentation from the DICOM data when obtaining the STL file. This process is observer-dependent and requires extensive anatomical knowledge. The accuracy of replicating the cardiac and vascular structural geometry must be validated. Any errors may have serious consequences if the model is created for detailed planning. Few studies have noted that 3D-printed heart models only complement the current diagnostic tools and should not be stand-alone tools for diagnosis or preoperative planning (2).
The future of 3D printing in cardiovascular surgery and interventions
A recent meta-analysis of 158 studies found that the most prominent limiting factors for 3D printing were precision, preparation time, and cost (54). To improve the efficiency and accuracy of 3D printing in the adult cardiovascular surgery field, a multidisciplinary team (MDT) involving cardiovascular surgeons, cardiac catheterization specialists, radiologists, and engineers is required. To determine if the use of 3D printing will become a routine clinical tool in the future, the clinical outcomes of patients who were treated with 3D printing must be followed up. Future multicenter randomized studies including more cases are warranted to investigate the clinical value and to confirm the benefits of 3D printing in this field.
However, 3D printing technology has been rapidly applied in the field of cardiovascular surgery in recent years. 3D bioprinting is a very impressive new development, has potential to develop fully functional heart construct that can integrate with native tissues rapidly. In the near future, cardiovascular surgeon will get the tailored valves, vessels or even entire heart from patients’ own cells by 3D bioprinting, which will open the door to a new era of personalized medicine (51,55,56).
Limitations
This review presents limitations. Firstly, all of included publications on 3D printing technology in cardiovascular surgery and intervention still consist of single case reports or small case series, and possess all the inherent limitations. Secondly, the sample size is too small to draw conclusions concerning the reliability of the 3D printing technologies in cardiovascular surgery and interventions. Finally, the outcome of mortality, morbidity and follow-up is not available from this analysis. Therefore, general conclusion from solid statistical analysis is lacking.
Conclusions
In conclusion, 3D printing is a promising technology, has been gradually more utilized in adult cardiovascular surgery and interventions and is helpful for preoperative planning, intraoperative orientation, and medical teaching. Despite its advantages, 3D printing in cardiovascular medicine can be accepted in selected cases. Indeed, clinical randomized control trials with large samples are needed to assess its values on the diagnostic tools and clinical outcomes.
Acknowledgments
Funding: None.
Footnote
Reporting Checklist: The authors have completed the PRISMA reporting checklist. Available at http://dx.doi.org/10.21037/jtd-20-455
Conflicts of Interest: All authors have completed the ICMJE uniform disclosure form (available at http://dx.doi.org/10.21037/jtd-20-455). The authors have no conflicts of interest to declare.
Ethical Statement: The authors are accountable for all aspects of the work in ensuring that questions related to the accuracy or integrity of any part of the work are appropriately investigated and resolved.
Open Access Statement: This is an Open Access article distributed in accordance with the Creative Commons Attribution-NonCommercial-NoDerivs 4.0 International License (CC BY-NC-ND 4.0), which permits the non-commercial replication and distribution of the article with the strict proviso that no changes or edits are made and the original work is properly cited (including links to both the formal publication through the relevant DOI and the license). See: https://creativecommons.org/licenses/by-nc-nd/4.0/.
References
- Chepelev L, Wake N, Ryan J, et al. Radiological Society of North America (RSNA) 3D printing Special Interest Group (SIG): guidelines for medical 3D printing and appropriateness for clinical scenarios. 3D Print Med 2018;4:11.
- Lau I, Sun Z. Three-dimensional printing in congenital heart disease: A systematic review. J Med Radiat Sci 2018;65:226-36. [Crossref] [PubMed]
- Bartel T, Rivard A, Jimenez A, et al. Medical three-dimensional printing opens up new opportunities in cardiology and cardiac surgery. Eur Heart J 2018;39:1246-54. [Crossref] [PubMed]
- Liberati A, Altman DG, Tetzlaff J, et al. The PRISMA statement for reporting systematic reviews and meta-analyses of studies that evaluate health care interventions: explanation and elaboration. J Clin Epidemiol 2009;62:e1-e34. [Crossref] [PubMed]
- Sommer K, Izzo RL, Shepard L, et al. Design Optimization for Accurate Flow Simulations in 3D Printed Vascular Phantoms Derived from Computed Tomography Angiography. Proc SPIE Int Soc Opt Eng 2017;10138. doi: 10.1117/12.2253711. [Crossref]
- Mashari A, Knio Z, Jeganathan J, et al. Hemodynamic Testing of Patient-Specific Mitral Valves Using a Pulse Duplicator: A Clinical Application of Three-Dimensional Printing. J Cardiothorac Vasc Anesth 2016;30:1278-85. [Crossref] [PubMed]
- Kalejs M, von Segesser LK. Rapid prototyping of compliant human aortic roots for assessment of valved stents. Interact Cardiovasc Thorac Surg 2009;8:182-6. [Crossref] [PubMed]
- Abdel-Sayed P, Kalejs M, von Segesser LK. A new training set-up for trans-apical aortic valve replacement. Interact Cardiovasc Thorac Surg 2009;8:599-601. [Crossref] [PubMed]
- Engelhardt S, Sauerzapf S, Preim B, et al. Flexible and comprehensive patient-specific mitral valve silicone models with chordae tendineae made from 3D-printable molds. Int J Comput Assist Radiol Surg 2019;14:1177-86. [Crossref] [PubMed]
- Spinelli D, Marconi S, Caruso R, et al. 3D printing of aortic models as a teaching tool for improving understanding of aortic disease. J Cardiovasc Surg (Torino) 2019;60:582-8. [Crossref] [PubMed]
- Premyodhin N, Mandair D, Ferng AS, et al. 3D printed mitral valve models: affordable simulation for robotic mitral valve repair. Interact Cardiovasc Thorac Surg 2018;26:71-6. [Crossref] [PubMed]
- Shirakawa T, Yoshitatsu M, Koyama Y, et al. 3D-printed aortic stenosis model with fragile and crushable calcifications for off-the-job training and surgical simulation. Multimed Man Cardiothorac Surg 2018;2018. [Crossref] [PubMed]
- Fan Y, Yang F, Cheung GSH, et al. Device Sizing Guided by Echocardiography-Based Three-Dimensional Printing Is Associated with Superior Outcome after Percutaneous Left Atrial Appendage Occlusion. J Am Soc Echocardiogr 2019;32:708-719.e1. [Crossref] [PubMed]
- Hachulla AL, Noble S, Guglielmi G, et al. 3D-printed heart model to guide LAA closure: useful in clinical practice? Eur Radiol 2019;29:251-8. [Crossref] [PubMed]
- Hosny A, Dilley JD, Kelil T, et al. Pre-procedural fit-testing of TAVR valves using parametric modeling and 3D printing. J Cardiovasc Comput Tomogr 2019;13:21-30. [Crossref] [PubMed]
- Zhang J, Ma W, Zhang W, et al. Three-Dimensional Printed Models-Guided Surgical Repair for Recurrent Coronary Artery Fistula. Ann Thorac Surg 2019;107:e161-3. [Crossref] [PubMed]
- Misra A, Walters HL, Kobayashi D. Utilisation of a three-dimensional printed model for the management of coronary-pulmonary artery fistula from left main coronary artery. Cardiol Young 2019;29:431-4. [Crossref] [PubMed]
- Shijo T, Shirakawa T, Yoshitatsu M, et al. Stent grafting simulation using a three-dimensional printed model for extensive aortic arch repair combined with coarctation. Eur J Cardiothorac Surg 2018;54:593-5. [Crossref] [PubMed]
- Faletti R, Gatti M, Cosentino A, et al. 3D printing of the aortic annulus based on cardiovascular computed tomography: Preliminary experience in pre-procedural planning for aortic valve sizing. J Cardiovasc Comput Tomogr 2018;12:391-7. [Crossref] [PubMed]
- Lee M, Moharem-Elgamal S, Beckingham R, et al. Evaluating 3D-printed models of coronary anomalies: a survey among clinicians and researchers at a university hospital in the UK. BMJ Open 2019;9:e025227. [Crossref] [PubMed]
- Sun X, Zhang H, Zhu K, et al. Curved section modeling-based three-dimensional printing for guiding septal myectomy. J Thorac Dis 2018;10:E535-E537. [Crossref] [PubMed]
- Gomes EN, Dias RR, Rocha BA, et al. Use of 3D Printing in Preoperative Planning and Training for Aortic Endovascular Repair and Aortic Valve Disease. Braz J Cardiovasc Surg 2018;33:490-5. [Crossref] [PubMed]
- Guo HC, Wang Y, Dai J, et al. Application of 3D printing in the surgical planning of hypertrophic obstructive cardiomyopathy and physician-patient communication. J Thorac Dis 2018;10:867-73. [Crossref] [PubMed]
- Ginty OK, Moore JM, Xu Y, et al. Dynamic Patient-Specific Three-Dimensional Simulation of Mitral Repair: Can We Practice Mitral Repair Preoperatively? Innovations (Phila) 2018;13:11-22. [Crossref] [PubMed]
- Bagur R, Cheung A, Chu MWA, et al. 3-Dimensional-Printed Model for Planning Transcatheter Mitral Valve Replacement. JACC Cardiovasc Interv 2018;11:812-3. [Crossref] [PubMed]
- El Sabbagh A, Eleid MF, Matsumoto JM, et al. Three-dimensional prototyping for procedural simulation of transcatheter mitral valve replacement in patients with mitral annular calcification. Catheter Cardiovasc Interv 2018;92:E537-E549. [Crossref] [PubMed]
- Aroney N, Markham R, Putrino A, et al. Three-dimensional printed cardiac fistulae: a case series. Eur Heart J Case Rep 2019. doi: 10.1093/ehjcr/ytz060. [Crossref]
- Sun X, Zhang H, Zhu K, et al. Patient-specific three-dimensional printing for Kommerell's diverticulum. Int J Cardiol 2018;255:184-7. [Crossref] [PubMed]
- Song H, Zhou Q, Zhang L, et al. Evaluating the morphology of the left atrial appendage by a transesophageal echocardiographic 3-dimensional printed model. Medicine (Baltimore) 2017;96:e7865. [Crossref] [PubMed]
- Hamatani Y, Amaki M, Kanzaki H, et al. Contrast-enhanced computed tomography with myocardial three-dimensional printing can guide treatment in symptomatic hypertrophic obstructive cardiomyopathy. ESC Heart Fail 2017;4:665-9. [Crossref] [PubMed]
- Hermsen JL, Burke TM, Seslar SP, et al. Scan, plan, print, practice, perform: Development and use of a patient-specific 3-dimensional printed model in adult cardiac surgery. J Thorac Cardiovasc Surg 2017;153:132-40. [Crossref] [PubMed]
- Vukicevic M, Puperi DS, Jane Grande-Allen K, et al. 3D Printed Modeling of the Mitral Valve for Catheter-Based Structural Interventions. Ann Biomed Eng 2017;45:508-19. [Crossref] [PubMed]
- Ho D, Squelch A, Sun Z. Modelling of aortic aneurysm and aortic dissection through 3D printing. J Med Radiat Sci 2017;64:10-7. [Crossref] [PubMed]
- Pracon R, Grygoruk R, Dzielinska Z, et al. Percutaneous occlusion of the left atrial appendage with complex anatomy facilitated with 3D-printed model of the heart. EuroIntervention 2016;12:927. [Crossref] [PubMed]
- Liu P, Liu R, Zhang Y, et al. The Value of 3D Printing Models of Left Atrial Appendage Using Real-Time 3D Transesophageal Echocardiographic Data in Left Atrial Appendage Occlusion: Applications toward an Era of Truly Personalized Medicine. Cardiology 2016;135:255-61. [Crossref] [PubMed]
- Pellegrino PL, Fassini G, DI, Biase M, et al. Left Atrial Appendage Closure Guided by 3D Printed Cardiac Reconstruction: Emerging Directions and Future Trends. J Cardiovasc Electrophysiol 2016;27:768-71. [Crossref] [PubMed]
- Ripley B, Kelil T, Cheezum MK, et al. 3D printing based on cardiac CT assists anatomic visualization prior to transcatheter aortic valve replacement. J Cardiovasc Comput Tomogr 2016;10:28-36. [Crossref] [PubMed]
- Gallo M, D'Onofrio A, Tarantini G, et al. 3D-printing model for complex aortic transcatheter valve treatment. Int J Cardiol 2016;210:139-40. [Crossref] [PubMed]
- Al Jabbari O, Abu Saleh WK, Patel AP, et al. Use of three-dimensional models to assist in the resection of malignant cardiac tumors. J Card Surg 2016;31:581-3. [Crossref] [PubMed]
- Hossien A, Gesomino S, Maessen J, et al. The Interactive Use of Multi-Dimensional Modeling and 3D Printing in Preplanning of Type A Aortic Dissection. J Card Surg 2016;31:441-5. [Crossref] [PubMed]
- Son KH, Kim KW, Ahn CB, et al. Surgical Planning by 3D Printing for Primary Cardiac Schwannoma Resection. Yonsei Med J 2015;56:1735-7. [Crossref] [PubMed]
- Otton JM, Spina R, Sulas R, et al. Left Atrial Appendage Closure Guided by Personalized 3D-Printed Cardiac Reconstruction. JACC Cardiovasc Interv 2015;8:1004-6. [Crossref] [PubMed]
- Schmauss D, Haeberle S, Hagl C, et al. Three-dimensional printing in cardiac surgery and interventional cardiology: a single-centre experience. Eur J Cardiothorac Surg 2015;47:1044-52. [Crossref] [PubMed]
- Dankowski R, Baszko A, Sutherland M, et al. 3D heart model printing for preparation of percutaneous structural interventions: description of the technology and case report. Kardiol Pol 2014;72:546-51. [Crossref] [PubMed]
- Maragiannis D, Jackson MS, Igo SR, et al. Functional 3D printed patient-specific modeling of severe aortic stenosis. J Am Coll Cardiol 2014;64:1066-8. [Crossref] [PubMed]
- Witschey WR, Pouch AM, McGarvey JR, et al. Three-dimensional ultrasound-derived physical mitral valve modeling. Ann Thorac Surg 2014;98:691-4. [Crossref] [PubMed]
- Jacobs S, Grunert R, Mohr FW, et al. 3D-Imaging of cardiac structures using 3D heart models for planning in heart surgery: a preliminary study. Interact Cardiovasc Thorac Surg 2008;7:6-9. [Crossref] [PubMed]
- Patel A, Cosman P, Desai S. Three-dimensional printing technology in surgery. Surgery Curr Res 2015;6:255.
- Guerrero M, Dvir D, Himbert D, et al. Transcatheter Mitral Valve Replacement in Native Mitral Valve Disease With Severe Mitral Annular Calcification: Results From the First Multicenter Global Registry. JACC Cardiovasc Interv 2016;9:1361-71. [Crossref] [PubMed]
- Farooqi KM, Sengupta PP. Echocardiography and three-dimensional printing: sound ideas to touch the heart. J Am Soc Echocardiogr 2015;28:398-403. [Crossref] [PubMed]
- Yoo SJ, Thabit O, Kim EK, et al. 3D printing in medicine of congenital heart diseases. 3D Print Med 2015;2:3.
- Giannopoulos AA, Steigner ML, George E, et al. Cardiothoracic Applications of 3-dimensional Printing. J Thorac Imaging 2016;31:253-72. [Crossref] [PubMed]
- Vukicevic M, Mosadegh B, Min JK, et al. Cardiac 3D Printing and its Future Directions. JACC Cardiovasc Imaging 2017;10:171-84. [Crossref] [PubMed]
- Martelli N, Serrano C, van den Brink H, et al. Advantages and disadvantages of 3- dimensional printing in surgery: A systematic review. Surgery 2016;159:1485-500. [Crossref] [PubMed]
- Qasim M, Haq F, Kang MH, Kim JH. 3D printing approaches for cardiac tissue engineering and role of immune modulation in tissue regeneration. Int J Nanomedicine 2019;14:1311-33. [Crossref] [PubMed]
- Lueders C, Jastram B, Hetzer R, et al. Rapid manufacturing techniques for the tissue engineering of human heart valves. Eur J Cardiothorac Surg 2014;46:593-601. [Crossref] [PubMed]